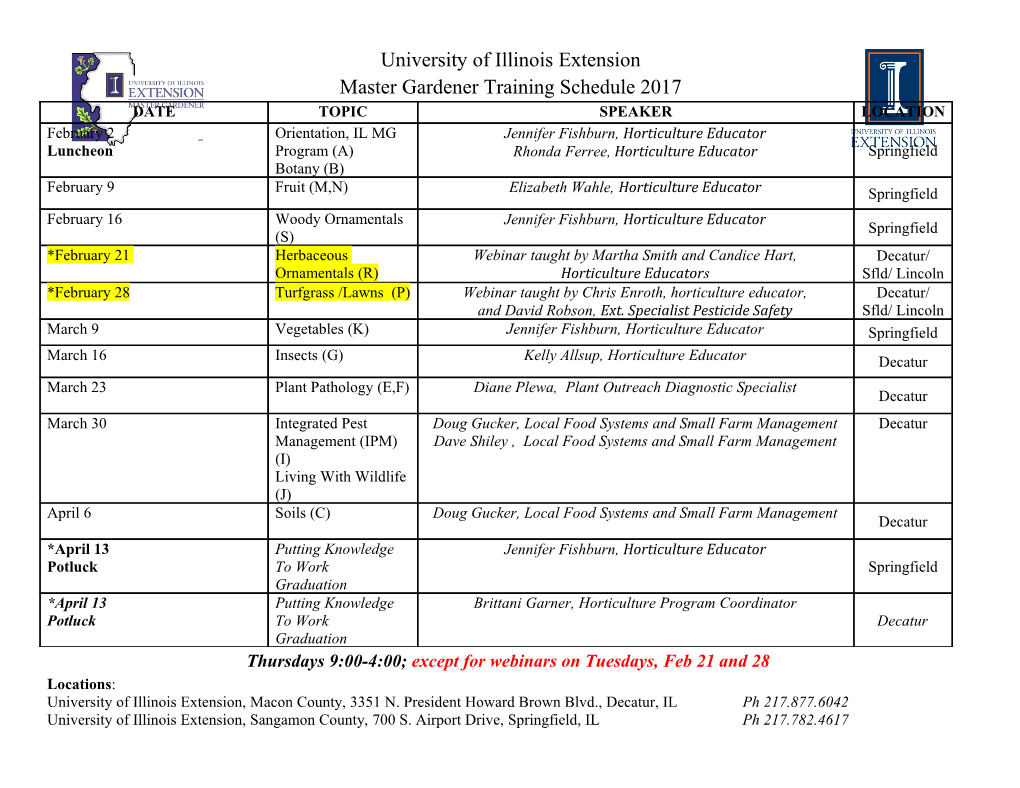
15 ENERGY PERSPECTIVES IN SWITZERLAND: THE POTENTIAL OF NUCLEAR POWER K. Foskolos, P. Hardegger In 2004, discussions were started in Switzerland concerning future of energy supply, including domestic electricity generation. On behalf of the Federal Office of Energy, PSI undertook a study to evaluate the potential of future nuclear technologies, covering electricity demand, with a time horizon up to 2050. It has been shown that nuclear power plants (NPPs) of the Third Generation, similar to the ones currently under construction in several other countries, built on the existing nuclear sites in Switzerland, have the potential to replace, at competitive costs, the existing nuclear plants, and even to cover (postulated) increases in electricity demand. Because of their late maturity (expected at the earliest around 2030), NPPs of the Fourth Generation, which are currently under development, cannot play a major role in Switzerland, since, with the exception of the Leibstadt NPP, all decisions regarding replacement of the current Swiss NPPs have to be taken before 2030. 1 INTRODUCTION Following the start of construction in Finland (Olkiluoto III), and the decision to construct a similar plant in Electricity production in Switzerland is practically France (Flamanville III), particular importance is CO -free. This is due to the high proportion of hydro 2 attributed to the European Pressurized (Water) and nuclear power (56% and 40%, respectively, in Reactor (EPR), a Generation III concept of about 2003) in the total electricity budget. Maintaining an 1600 MW , developed by Framatome ANP, and electricity mix with low CO emissions will be essential e 2 based on proven, standardized French (N4) and – at least in the mid-term – in achieving current German (Konvoi) reactor concepts. climate protection objectives, e.g. those of the Kyoto Protocol. Consequently, in 2004, the Swiss Federal Office of Energy initiated a broad study to assess the Double Containment potential of the different energy carriers to provide a with Ring Space Venting Melt spread secure energy supply, while continuing to satisfy the surface Swiss environmental criteria. PSI has provided Containment contributions to this study in regard to renewable Heat Removal System energies and new nuclear power plants. The main findings for the nuclear energy part of the report are summarized in this paper. EPR 2 CURRENT NUCLEAR ENERGY TECHNOLOGIES Integrated 4-fold Flooding Pool / Sump Safety The development of nuclear technology can be Redundancy conveniently subdivided into generations. Prototype and demonstration plants from the 50s and 60s belong to Generation I. Some technological lines, Fig. 1: EPR and its main safety-related innovations. mainly water-cooled reactors, became commercially successful in the 70s and 80s, and today constitute In the field of gas-cooled reactors, there are currently the bulk of the world’s current nuclear park: these developments of concepts involving a direct helium reactors belong to Generation II. More than cycle, and helium turbines. In South Africa, a decision 10 000 reactor·years of cumulative operational to start construction of the PBMR, a gas-cooled, experience, together with the lessons learned from the pebble-bed modular reactor, is expected shortly. two major nuclear accidents at Three Mile Island and Recent press articles have underlined the importance Chernobyl, have led to a significantly improved safety of the EPR as a potential replacement for existing level for the Generation II reactors. reactors in Switzerland. Although this hypothesis is New safety and system approaches were developed plausible, no concrete decisions have yet been made in the 90s, and integrated into the advanced reactor regarding any particular technology line. Such concepts comprising Generation III. Generation III is decisions necessitate extensive and time-consuming characterized by the innovativeness of the evaluations of different criteria, including existing approaches; for example, those integrating passive operational experience. It may be assumed, however, safety systems and inherent safety mechanisms. that in the case of a nuclear option for the Those allowing for shorter construction times, lower replacement of existing plants, Light Water Reactor O&M costs and increased efficiency, are often (LWR) technology will remain the first consideration. designated Generation III+ reactors. However, the distinction between the two is not unambiguous, and the two concepts are therefore taken together here. 16 3 FUTURE NUCLEAR ENERGY including, in the event of core damage, a very low TECHNOLOGIES: GENERATION IV likelihood of significant radioactivity releases to the environment, thus eliminating the need for off-site To meet future energy needs, ten countries emergency response. Finally, the Generation IV (Argentina, Brazil, Canada, France, Japan, the reactors aim at drastically reducing the proliferation Republic of Korea, the Republic of South Africa, risk of weapon-grade materials, and provide high Switzerland, the United Kingdom, the United States) levels of protection against acts of terrorism. and one organisation (Euratom) have agreed on an international cooperative research framework for an More than 100 experts from the GIF partner countries advanced generation of nuclear reactor: Generation have evaluated the 100 or more concepts for IV. The eleven partners assembled for the first time in Generation IV systems proposed by the worldwide 2001 to form the Generation IV International Forum R&D community, and selected the six most promising (GIF). Though recognized as an organisation with a lines: the Gas-Cooled Fast Reactor, the Lead-Cooled long-term perspective, at least some of the Fast Reactor, the Molten Salt Reactor, the Sodium- Generation IV nuclear energy systems could be Cooled Fast Reactor, the Supercritical Water-Cooled available by the year 2030, the time at which many of Reactor, and the Very-High-Temperature Reactor. the currently operating nuclear power plants in the Table 1 provides a summary overview of these six world will be at, or near to, the end of their operating Generation IV systems. In assembling the data, the lifetimes. two extra important considerations of electricity generation ― hydrogen and process heat production, The points of focus for the development of new and actinide management ― were addressed. In this systems are sustainability, economics, safety and process, the range of national priorities and interests reliability, resistance to proliferation, and physical of the individual GIF countries has been taken into protection. The Generation IV systems should meet account. the clean-air objectives, and promote effective fuel utilization. The issues of minimization of nuclear Common trends for most of the selected systems are waste, and reduction of long-term burden of the use of a closed, fast-neutron nuclear fuel cycle, monitoring for future generations, are also being including reprocessing, recycling and transmutation of addressed. The systems target clear life-cycle cost actinides, and the high operating temperatures of the advantages over other energy sources, at a reactor coolant, ensuring high thermal efficiency and comparable level of financial risk. In addition, they the possibility of efficient process-heat applications. prescribe excellence in terms of safety and reliability, Table 1: Overview of the Generation IV Systems. Neutron- Fuel Size System Abbrev. Coolant Temperature Pressure Fuel Use spectrum cycle (MWe) Gas-cooled U-238 electricity closed, fast GFR fast helium 850°C high & 288 & in-situ reactor MOX hydrogen 50- Lead-cooled U-238 150, electricity fast reactor closed, LFR fast Pb-Bi 550-800°C low & 300- & (liquid-metal regional MOX 400, hydrogen cooled ) 1200 electricity Molten salt fluoride UF in closed, MSR epithermal 700-800°C low 6 1000 & reactor salts salt in-situ hydrogen Sodium- U-238 300- cooled fast SFR fast sodium 550°C low & closed electricity 1500 reactor MOX Supercritical water- thermal/ open (th) SCWR water 510-550°C very high UO2 1500 electricity cooled fast closed (f) reactor Very high hydrogen temperature VHTR thermal helium 1000°C high UO2 open 250 & gas reactor electricity 17 The boundary conditions for the possible replacement A transition to fast neutron spectra, and thereby an of the nuclear power reactors in Switzerland are not increase in fuel utilization by a factor 50-100, will favourable to any of the Generation IV concepts. become possible only with Generation IV systems. Assuming that the existing NPPs will be replaced at Reprocessing is still a controversial issue. The new the end of their technical lifetimes, and that licensing, Swiss Atomic Law imposed a 10-year moratorium on planning and construction of new NPPs would require reprocessing, starting in 2006. Recycling through at least ten years, it is evident that Generation IV reprocessing of spent fuel in any case saves 15-20% systems will not be commercially available, or of the uranium, and reduces the final storage capacity. adequately proven, at the time the decision for the replacement of Beznau I, Beznau II, Mühleberg and Waste disposal is progressing worldwide: several Gösgen has to be taken: all before 2030. The use of a repositories for low-level and short-lived/medium-level Generation IV system to replace Leibstadt (around waste are already in operation, and first repositories 2045) is theoretically possible, provided that the most for high-level waste and spent fuel are under advanced systems at that
Details
-
File Typepdf
-
Upload Time-
-
Content LanguagesEnglish
-
Upload UserAnonymous/Not logged-in
-
File Pages10 Page
-
File Size-