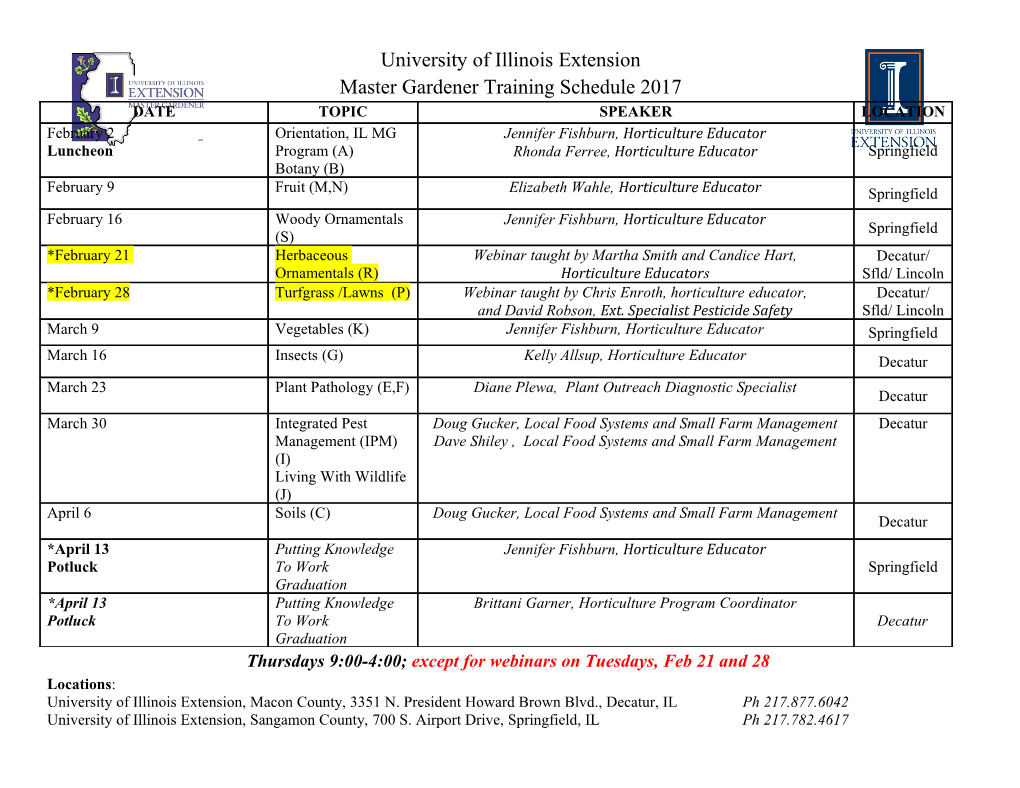
JGeod DOI 10.1007/s00190-011-0509-4 ORIGINAL ARTICLE Orbit determination of the Lunar Reconnaissance Orbiter Erwan Mazarico · D. D. Rowlands · G. A. Neumann · D. E. Smith · M. H. Torrence · F. G. Lemoine · M. T. Zuber Received: 17 March 2011 / Accepted: 13 August 2011 © Springer-Verlag 2011 Abstract We present the results on precision orbit deter- Keywords Moon · Orbit determination · Gravity · mination from the radio science investigation of the Lunar Altimetric crossover · Geodesy Reconnaissance Orbiter (LRO) spacecraft. We describe the data, modeling and methods used to achieve position knowl- edge several times better than the required 50–100 m (in total 1 Introduction position), over the period from 13 July 2009 to 31 January 2011. In addition to the near-continuous radiometric track- 1.1 Objectives ing data, we include altimetric data from the Lunar Orbiter Laser Altimeter (LOLA) in the form of crossover measure- The Lunar Reconnaissance Orbiter (LRO) mission (Chin ments, and show that they strongly improve the accuracy of et al. 2007) was designed to provide a comprehensive (global) the orbit reconstruction (total position overlap differences and detailed (high-resolution) survey of the Moon, in antic- decrease from ∼70 m to ∼23 m). To refine the spacecraft ipation of the needs of future orbital and landed exploration trajectory further, we develop a lunar gravity field by com- missions. The payload consists of seven instruments (Von- bining the newly acquired LRO data with the historical data. drak et al. 2010), some of which have very high spatial resolu- The reprocessing of the spacecraft trajectory with that model tion: better than 50 cm for the Lunar Reconnaissance Orbiter shows significantly increased accuracy (∼20 m with only the Camera (LROC) system (Robinson et al. 2010), ∼5-m foot- radiometric data, and ∼14 m with the addition of the altimet- prints and 10-cm vertical precision for the Lunar Orbiter ric crossovers). LOLA topographic maps and calibration data Laser Altimeter (LOLA, Smith et al. 2010a); 320 × 160 m from the Lunar Reconnaissance Orbiter Camera were used field-of-view for the Diviner Lunar Radiometer Experiment to supplement the results of the overlap analysis and demon- (DLRE, Paige et al. 2010); 30-m zoom resolution for the Min- strate the trajectory accuracy. iature Radio Frequency Technology Demonstration (Mini- RF, Nozette et al. 2010). The LRO datasets will ultimately be combined in a single reference frame based on the LOLA- Electronic supplementary material The online version of this defined geodetic grid, which requires accurate and consis- article (doi:10.1007/s00190-011-0509-4) contains supplementary tent trajectories for geolocation. As such, the LRO mission material, which is available to authorized users. defined a position knowledge requirement: 50–100 m total E. Mazarico · D. E. Smith · M. T. Zuber position and 1-m radially (Vondrak et al. 2010). Massachusetts Institute of Technology, Cambridge, USA Our goal is to report on the current status of the LRO precision orbit determination (POD). To determine precision E. Mazarico (B) · D. D. Rowlands · G. A. Neumann · D. E. Smith · M. H. Torrence · F. G. Lemoine orbits, the LRO trajectory from 13 July 2009 to 31 January NASA Goddard Space Flight Center, Planetary Geodynamics 2011 was reconstructed continuously, except for short gaps Laboratory, Greenbelt, USA near spacecraft maneuvers. As described below, the quality e-mail: [email protected] of the orbits is assessed primarily through overlap analysis, M. H. Torrence i.e., the consistency of trajectory segments when processed S.G.T. Inc., Greenbelt, USA in two consecutive time periods. 123 E. Mazarico et al. To obtain the most precise orbits for LRO, we have devel- the spacecraft commissioning phase was initiated on 27 June oped a gravity model based on the historical tracking data 2009. The commissioning orbit was a quasi-frozen ∼30 × (Mazarico et al. 2010) and further tuned with data from LRO. 200 km polar orbit (i ∼ 90◦), with its periapsis near the lunar This intermediary model is not optimized for geophysical south pole. purposes and is intended to be used only to improve the LRO The instrument commissioning phase began on 3 July position knowledge. The upcoming Gravity Recovery and 2009. LOLA was initially turned on for 2 days at that time Interior Laboratory (GRAIL) mission (Zuber et al. 2011), and started continuous data collection on 13 July 2009. Since scheduled for launch in September 2011, should provide a then, it has operated continuously except for the monthly sta- lunar gravity field with far greater accuracy than what is pos- tion-keeping (SK) maneuvers, and a February 2010 safehold sible to achieve from analysis of LRO tracking data. event. On 15 September 2009, the spacecraft transitioned to its 1.2 Previous work nominal 2-h period mapping orbit, with lower eccentricity and an average altitude of 50 km. Because of gravitational In the recent era of lunar exploration, the Clementine space- perturbations, the orbit eccentricity and argument of periap- craft was the first to provide the data to determine the Moon’s sis naturally evolve, and monthly station-keeping maneuvers gravity field to relatively high spatial resolutions (l = 70, are necessary to preserve the mapping orbit (Houghton et al. Lemoine et al. 1997). The subsequent Lunar Prospector, 2007). The eccentricity is 0.0054 ± 0.0019, and never more with its lower orbit, in particular during the extended mis- than 0.010, with the spacecraft altitude generally between 35 sion (<50 km), enabled features as small as ∼40 km (l = and 65 km (referenced to a 1,737.4 km sphere). After 1 year 150−165) to be resolved (Konopliv et al. 2001). The recent of the nominal Exploration mission, dedicated to the survey JAXA SELENE mission consisted of three spacecraft: the of the polar regions and of 50 sites selected for NASA’s Con- main orbiter Kaguya, in a 100 km-altitude circular orbit, stellation human space flight program, the LRO mission was and two sub-satellites which participated in a VLBI exper- extended for 2 years by the NASA Science Mission Direc- iment. One of those sub-satellites also provided relay capa- torate and began its Science mission. bility for the radio signals, between ground stations and the In addition to the monthly SK maneuvers, smaller angular main orbiter. This enabled radiometric tracking of the far- momentum desaturation events (δH) generally occur twice side for the first time and led to great improvements in the a month: once just before an SK maneuver, and the other determination of the lunar farside gravity field (Namiki 2009; about 2 weeks later. When the Sun-orbit geometry is favor- Matsumoto et al. 2010; Goossens et al. 2011). able (large β angle1), enough power can be generated with the solar panel held fixed, which reduces the angular momen- 1.3 Overview tum accumulated in the reaction wheels and can allow a δH to be skipped (e.g., in July 2010). After a brief summary of the LRO mission as it relates to Other kinds of maneuvers are less frequent (yaw flip every the presented work, we describe the tracking data types used 6 months, at β ∼ 0◦ to keep the single solar panel oriented here: radiometric Doppler and range, and altimetric cross- towards the Sun), or unique (phasing maneuver to observe overs from LOLA. The orbit determination process is then the LCROSS impact in October 2009). Table S1 lists the described in detail, followed by a discussion of the various maneuvers of the nominal and science missions. steps in the current-best orbit reconstruction: radiometric- The orbital maneuver plan leaves LRO in free flight for only orbits, the addition of the altimeter data, and the inver- ∼14-day periods, which is desirable for orbit determination sion of a new LRO-tuned gravity field (LLGM-1, LRO Lunar and gravity field estimation. It enables orbit reconstruction Gravity Model), which is used to converge the final trajectory. to be executed over extended time spans, thereby facilitat- Finally, orbit overlap analyses are supplemented by results ing the recovery of long-period perturbations due to the long using independent data: LOLA topographic maps of the polar wavelengths of the gravity field (i.e., low-degree spherical regions and LROC calibration information. harmonic expansion coefficients, especially the zonal and resonant terms). It also improves the contribution of altimet- 2Data ric crossovers to the solution, by reducing the number of effectively independent spacecraft initial states that need to 2.1 Mission summary be estimated. The regularity of the spacecraft SK maneuvers leads to The LRO spacecraft was launched at 22:32 UTC on 18 June a natural partition of the mission into monthly periods, 2009 from Cape Canaveral Launch Complex 41, and entered lunar orbit on 23 June 2009. Five maneuvers designed to 1 Thebetaangle(β) is the viewing angle of the Sun from the orbit, gradually circularize the initial eccentric orbit followed, and i.e., the angle between the orbit plane and the spacecraft–Sun vector. 123 LRO orbit determination or “phases”, each lasting for approximately one lunation atic because they usually provide the strongest control of the (28 days). In the time period presented here (13 July 2009 spacecraft orbit (Table S2). Early in the mission, the Doppler to 31 January 2011), there are 21 phases in total. Commis- data collected by the White Sands station were time-biased by sioning, which lacks SK maneuvers, is divided into three 1/76th of a second (around −13.16 ms); this was corrected phases of equivalent duration (CO_01 to CO_03). The nom- on 19 October 2009. The Range data from all stations are inal mission is split into 13 phases (NO_01 to NO_13), and also affected by timing biases.
Details
-
File Typepdf
-
Upload Time-
-
Content LanguagesEnglish
-
Upload UserAnonymous/Not logged-in
-
File Pages15 Page
-
File Size-