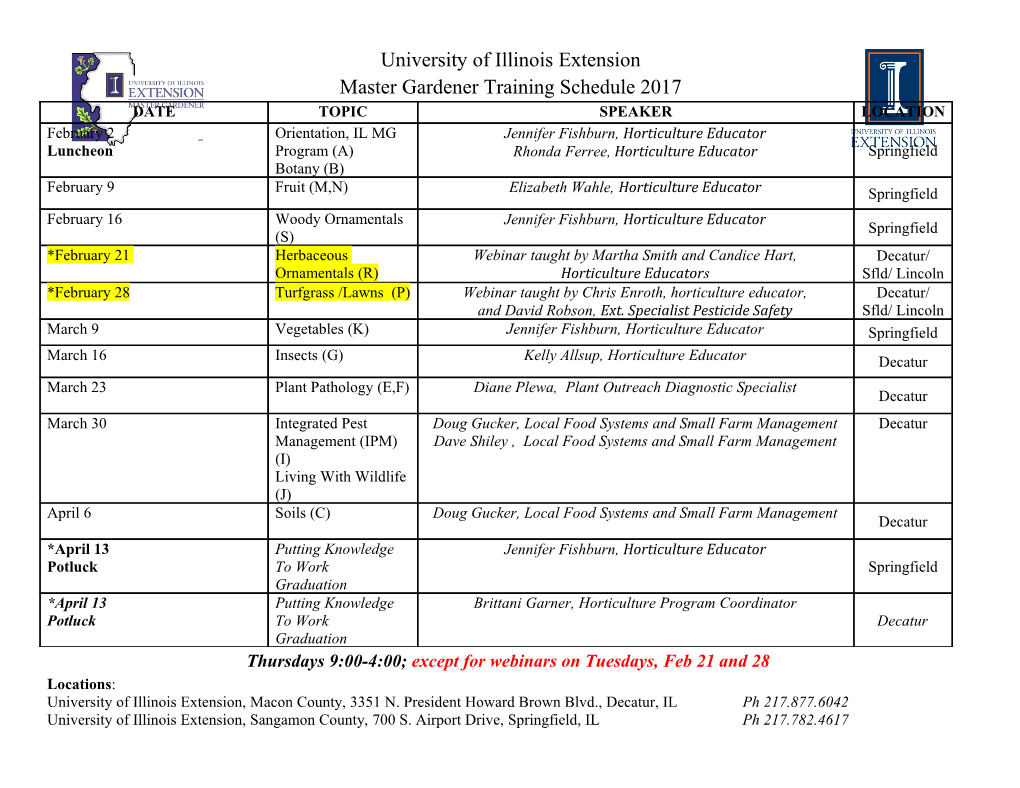
Quantitative Fluorescence Microscopy of Protein Dynamics in Living Cells Shehu Mustapha Ibrahim The work described in this thesis was performed at the Department of Pathology of the Josephine Nefkens Institute, Erasmus MC, Rotterdam and Department of Biophysics and Cell Biology, University of Debrecen, Medical and Health Science Centre, Hungary. Quantitative Fluorescence Microscopy of Protein Dynamics in Living Cells Kwantitatieve Fluorescentiemicroscopie van Eiwitdynamiek in Levende Cellen Proefschrift ter verkrijging van de graad van doctor aan de Erasmus Universiteit Rotterdam op gezag van de rector magnificus Prof. dr. S.W.J. Lamberts en volgens besluit van het College voor Promoties. De openbare verdediging zal plaatsvinden op donderdag 21 december 2006 om 09:00 uur door Shehu Mustapha Ibrahim Geboren te Ilorin, Nigeria Promotiecommissie Promotor: Prof.dr. J.H.J. Hoeijmakers Overige leden: Prof.dr.ir. J. Trapman Prof.dr. J. Szöllősi Dr. R. Krams Copromotores: Dr. A.B. Houtsmuller Dr. G. Vereb Contents Abbreviations 7 Chapter 1 General Introduction 9 Chapter 2 Light Driven Dynamics of GFP Fluorescence 49 Emission in Living Cells Chapter 3 Dynamics of Nuclear Proteins: 73 Complementarities of Fluorescence Correlation Microscopy and Photobleaching in Intra-Cellular Mobility Measurements Chapter 4 In the Absence of DNA Damage the Nuclear Mobility 109 of Most Nucleotide Excision Factors is Mainly Determined by their Molecular Size Chapter 5 Recruitment of the Nucleotide Excision 131 Endonuclease XPG to sites of UV-induced Damage Depends on Functional TFIIH Chapter 6 Translational Mobility of EGF Receptor Fusion 169 Proteins over Short and Long Distances – Complementarity of Fluorescence Correlation Microscopy and FRAP in Cellular Diffusion Measurements Chapter 7 Cholesterol-dependent Clustering of IL-2Rα 223 and its Colocalization with HLA and CD48 on T Lymphoma Cells suggests their Functional Association with Lipid Summary 249 Samenvating 253 Acknowledgements 257 List of publications 259 Curriculum Vitae 261 Abbreviations AOM Acousto-optical modulator AR Androgen receptor ARE Androgen response element BCIP Bromo-4-chloro-3-indolyl phosphate CAF I Chromatin assembly factor I CHO Chinese hamster ovary CLSM Confocal laser scanning microscopes CPD Cyclobutane pyrimidine dimers CS Cockayne syndrome DBD DNA binding domain DBD DNA-binding domain DM Dichroic mirror EBFP Enhanced blue fluorescent protein ECFP Enhanced cyan fluorescent protein EGF Epidermal growth factor EGFP Enhanced green fluorescent protein EGFR Epidermal growth factor receptor ERCC Excision repair cross complementing protein ESPT Excited state proton transfer EYFP Enhanced yellow fluorescent protein FCM Fluorescence correlation microscopy FCS Fluorescence correlation spectroscopy FP Fusion protein FRAP Fluorescence recovery after photobleaching FRET Fluorescence resonance energy transfer FWHM Full width half-maximum GFP Green fluorescence protein GG-NER Global genome NER IC Internal conversion IL-2 Interleukin-2 IL-2R Interleukin-2 receptor ISC Intersystem crossing LBD ligand-binding domain NA Numerical aperture NBT Nitro blue tetrazolium NER Nucleotide excision repair NLS Nuclear localisation signal NTD N-terminal transactivation domain PBS Phosphate-buffered saline PMT Photomultiplier tube RCFPs Reef coral fluorescent proteins ROI Region of interest RPA Replication Protein A RT Room temperature S0 Electronic ground state S1, S2 and so on Singlet excited energy states SNOM Scanning near-field optical microscopy SR Steroid receptor T1 Excited triplet state TC-NER Transcription-coupled NER TIR Total internal reflection TrfR Transferrin receptor TTD Trichothiodystrophy UV Ultraviolet wt-GFP Wild-type green fluorescent protein XP Xeroderma pigmentosum XPA Xeroderma pigmentosum A XPB Xeroderma pigmentosum B XPC Xeroderma pigmentosum C XPD Xeroderma pigmentosum D XPE Xeroderma pigmentosum E XPF Xeroderma pigmentosum F XPG Xeroderma pigmentosum G XPV Xeroderma pigmentosum V Chapter 1 General Introduction In fluorescence microscopy, the ability of fluorescent molecules to emit light of specific wavelengths following the absorption of light of shorter wavelengths is utilized to observe the molecules directly or to indicate the position of fluorescently tagged target molecules in a cell. Recent advances in laser and computer technologies have lead to the availability of commercial confocal laser scanning microscopes (CLSM) thereby making fluorescence microscopy widely accessible. Furthermore, the development of genetically encoded fluorescent proteins have lead to advances in the analytical techniques used for the evaluation of protein localization, dynamics and interactions in the cellular environment. A perfect fluorescence assay is one, which could monitor every protein directly and map its behaviour in time. However, at present no single imaging modality can assess all aspects of the complicated processes a protein undergoes in carrying out its functions in the living cell. Proteins are mostly observed indirectly with the use of fluorescent tags, which are assumed not to interfere with the behaviour of the target protein being studied. High-resolution digital imaging of fusion proteins (FP) enables protein localization and colocalization to be monitored in time. More sophisticated quantitative techniques, such as fluorescence recovery after photobleaching (FRAP) and fluorescence correlation spectroscopy (FCS) are used to study protein dynamics and protein-protein interactions. Although FRAP is a bulk assay method and FCS is more effective when only a few molecules are monitored at a time, the complementary use of both methods provides a more accurate insight into the movement of proteins and their interactions with cellular components in living cells. Most fluorescence assay methods rely on fluorescence changes to quantify the dynamics leading to these changes. Therefore an accurate understanding of protein behaviour does not only require the use of more than one assay technique where Chapter 1 10 necessary but also a knowledge of the photodynamic properties of the fluorescent tag being used, for a proper interpretation of the fluorescence data generated by these techniques. In this thesis, the efficacies of various fluorescence microscopy techniques in the analysis of protein localizations and dynamics in living cells are examined. In particular the complementarities of these methods have been investigated and the fluorescence behaviour of the enhanced green fluorescent protein (EGFP) considered in the interpretation of the fluorescence data. In the following paragraphs the basic principles of fluorescence microscopy will be discussed after a short history of the optical microscope. The green fluorescence proteins (GFPs) and their properties that make them convenient fluorescent probes for in vivo and in-situ analysis of proteins will be presented followed by a description of various fluorescence assay methods. Finally, a description of the proteins studied in this thesis will be given, followed by an outline of the thesis. 1.1 Short history of the optical microscope The magnifying and light focussing abilities of lenses have been observed as early as the first century when rudimentary lenses formed from transparent crystals were used to focus sunlight on dry materials to make fire. It was, however, not until the Renaissance when the first simple microscope was constructed. The humble 10x magnification achieved by the assembly, consisting of a magnifying lens and a plate situated at either ends of a tube, was then unprecedented as it made visible the fascinating details of objects. This inspired the Dutch spectacle maker Zacharias Janssen and his son Hans in 1590 to experiment with several lenses in a tube to enhance the achievable magnification thereby constructing the forerunner of the compound microscope and of the telescope. Galileo in 1609 worked out the principles of lenses and made an improved instrument 11 General Introduction equipped with a focussing device. Later, Antonie van Leeuwenhoek (1632-1723), credited with founding the field of microscopy, built microscopes using tiny lenses of very high magnifications to make his pioneering studies on bacteria, yeast, plants and the circulation of blood corpuscles in capillaries. Ingenious improvements to the light microscope continued through the nineteenth century. High quality lenses were being used in the optical elements and illumination systems were introduced. However, with the introduction of oil-immersion objectives at the end of the nineteenth century, the optical microscope reached its limits of resolution imposed by the diffraction of light. As discovered by Ernest Abbe in 1878, the best resolution attainable with the use of white light of average wavelength of 0.55 µm is 0.275 µm. It was thus realised that better resolutions could only be achieved by the use of a source of illumination with a much shorter wavelength. (The shortest wavelength of visible light, 0.4 µm could yield a resolution of 0.2µm.) In 1904, Köhler and Moritz von Rohr designed the ultraviolet microscope which was illuminated by ultraviolet radiation generated through a cadmium arc and used lenses fabricated from fused quartz. Operating in the shortest wavelength range of visible light, the microscope enabled images of high resolutions to be made. Inevitably, fluorescence was observed, thus establishing the foundation for ultraviolet and fluorescence microscopy. In 1934 Frits Zernike introduced the phase-contrast technique, which
Details
-
File Typepdf
-
Upload Time-
-
Content LanguagesEnglish
-
Upload UserAnonymous/Not logged-in
-
File Pages261 Page
-
File Size-