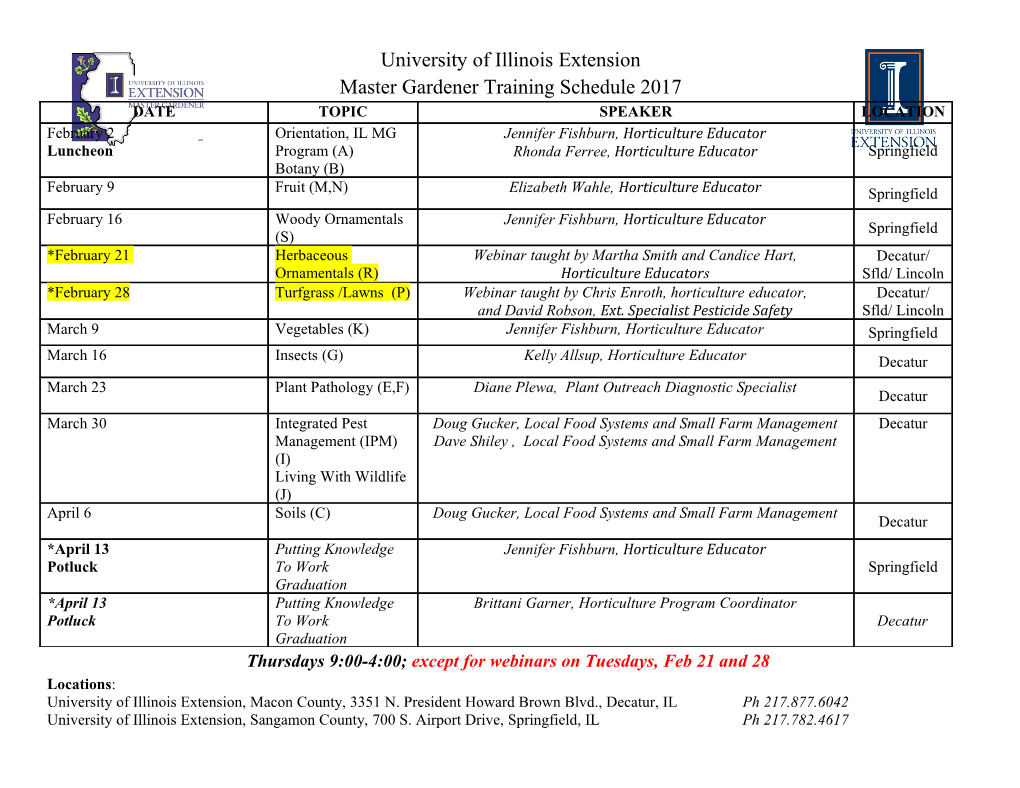
bioRxiv preprint doi: https://doi.org/10.1101/042705; this version posted March 10, 2016. The copyright holder for this preprint (which was not certified by peer review) is the author/funder, who has granted bioRxiv a license to display the preprint in perpetuity. It is made available under aCC-BY 4.0 International license. 1 Functional metagenomics using Pseudomonas putida expands the known diversity of 2 polyhydroxyalkanoate synthases and enables the production of novel polyhydroxyalkanoate 3 copolymers 4 5 Jiujun Cheng and Trevor C. Charles* 6 7 Department of Biology and Centre for Bioengineering and Biotechnology, University of Waterloo, 8 Waterloo, Ontario, Canada N2L 3G1 9 10 *Corresponding author: 11 Dr. Trevor C. Charles 12 Department of Biology, University of Waterloo, Waterloo, Ontario, Canada N2L 3G1. 13 E-mail: [email protected] 14 15 1 bioRxiv preprint doi: https://doi.org/10.1101/042705; this version posted March 10, 2016. The copyright holder for this preprint (which was not certified by peer review) is the author/funder, who has granted bioRxiv a license to display the preprint in perpetuity. It is made available under aCC-BY 4.0 International license. 16 17 Abstract 18 Bacterially produced biodegradable polyhydroxyalkanoates with versatile properties can be achieved 19 using different PHA synthase enzymes. This work aims to expand the diversity of known PHA 20 synthases via functional metagenomics, and demonstrates the use of these novel enzymes in PHA 21 production. Complementation of a PHA synthesis deficient Pseudomonas putida strain with a soil 22 metagenomic cosmid library retrieved 27 clones expressing either Class I, Class II or unclassified 23 PHA synthases, and many did not have close sequence matches to known PHA synthases. The 24 composition of PHA produced by these clones was dependent on both the supplied growth substrates 25 and the nature of the PHA synthase, with various combinations of SCL- and MCL-PHA. These data 26 demonstrate the ability to isolate diverse genes for PHA synthesis by functional metagenomics, and 27 their use for the production of a variety of PHA polymer and copolymer mixtures. 28 Keywords: 29 Functional metagenomics; soil metagenomic library; polyhydroxyalkanoate (PHA); bioplastics; 30 polyhydroxyalkanoate synthase; Pseudomonas putida; Sinorhizobium meliloti 31 2 bioRxiv preprint doi: https://doi.org/10.1101/042705; this version posted March 10, 2016. The copyright holder for this preprint (which was not certified by peer review) is the author/funder, who has granted bioRxiv a license to display the preprint in perpetuity. It is made available under aCC-BY 4.0 International license. 32 Introduction 33 Polyhydroxyalkanoates (PHAs) are natural polyesters biosynthesized by a variety of bacteria 34 under unbalanced growth conditions. They serve as reserves of carbon and reducing power and aid 35 in survival during starvation or stress conditions (Verlinden et al. 2007). These biodegradable and 36 environmentally friendly polymers can be used as alternative materials to conventional 37 petrochemical-based plastics. PHAs are classified into short-chain-length (SCL, C3-C5) and 38 medium-chain-length (MCL, ≥C6), and copolymers (SCL + MCL) based on the number of carbon 39 atoms per monomer. MCL-PHA is generally more useful than SCL-PHA due to it being less brittle 40 and more flexible. To produce PHA with versatile properties cost-effectively, strategies have 41 involved mining new PHA synthase enzymes (PhaC), engineering PhaC proteins and modifying the 42 metabolic pathways of the production strains (Keshavarz and Roy 2010; Schallmey et al. 2011; 43 Cheema et al. 2012; Park et al. 2012; Tripathi et al. 2013; Meng et al. 2014). 44 PHA molecules consist of over 150 possible constituent monomers of hydroxyl fatty acids 45 (Meng et al. 2014) (Steinbüchel and Lütke-Eversloh 2003). PHA synthase enzymes catalyze the 46 joining of the hydroxyl group of one monomer with the carboxyl group of another by an ester bond 47 to form PHA polymers. The monomeric composition of PHA is determined primarily by PhaC, 48 although the available carbon source and metabolic pathways also influence the properties of PHA 49 (Verlinden et al. 2007). PhaC proteins are grouped into four classes based on amino acid sequence, 50 substrate specificity and subunit composition (Rehm 2003). Class I and II PhaC consist of one 51 subunit (PhaC), but those in Class III and IV are composed of two subunits (PhaC+PhaE and 52 PhaC+PhaR, respectively). Class I and IV PhaCs synthesize SCL-PHA whereas Class II polymerize 53 MCL-PHA. Class III PhaCs can synthesize both SCL and MCL monomers. 3 bioRxiv preprint doi: https://doi.org/10.1101/042705; this version posted March 10, 2016. The copyright holder for this preprint (which was not certified by peer review) is the author/funder, who has granted bioRxiv a license to display the preprint in perpetuity. It is made available under aCC-BY 4.0 International license. 54 The key Class I PHA synthesis pathway genes include phaC (or phbC), phaA (or phbA) and 55 phaB (or phbB). The three genes are often, but not always, clustered in a single operon. The 56 acetoacetyl-CoA reductase (EC 2.3.1.9) encoded by the phaA and β-ketothiolase (EC 1.1.1.36) 57 encoded by the phaB convert acetyl-CoA to (R)-3-hydroxybutyryl-CoA (3HB-CoA) through 58 acetoacetyl-CoA. The Class-I PhaC (EC 2.3.1.-) then polymerizes 3HB-CoA into 59 polyhydroxybutyrate (PHB) (Rehm 2003). The Class II pha cluster is well conserved in 60 Pseudomonas and consists of two PHA synthase genes (phaC1 and phaC2) flanking a PHA 61 depolymerase gene (phaZ), and phaD encoding a transcriptional activator of pha genes. Phasin- 62 encoding phaF and phaI gene are transcribed divergently to other pha genes. MCL monomers ((R)- 63 3-hydroxylacyl-CoA) are derived from β-oxidation of fatty acids or fatty acid de novo synthesis from 64 unrelated carbon sources (Tortajada et al. 2013). 65 Traditional culture-based strategies for obtaining new biocatalysts are limited by the inability 66 to cultivate the majority of environmental microbes. While sequence-based metagenomics can 67 identify genes homologous to those present in available sequence databases, it is difficult to reliably 68 predict the function of truly new genes through homology-based analysis. In contrast, functional 69 metagenomics involves the construction of gene libraries from microbial community genomic DNA 70 and functional screening for novel enzymes of interest or potential industrial applications (Simon 71 and Daniel 2011). Functional metagenomics has the potential to identify truly novel sequences for a 72 given function. 73 In previous work, functional metagenomics was used to isolate new Class I PhaC from soil 74 metagenomic clones by Nile red staining and phenotypic screening in α-Proteobacteria 75 Sinorhizobium meliloti (Schallmey et al. 2011). In another study, phaC genes encoding both Class I 76 and II PhaC proteins were PCR amplified from oil-contaminated soil library clones (Cheema et al. 4 bioRxiv preprint doi: https://doi.org/10.1101/042705; this version posted March 10, 2016. The copyright holder for this preprint (which was not certified by peer review) is the author/funder, who has granted bioRxiv a license to display the preprint in perpetuity. It is made available under aCC-BY 4.0 International license. 77 2012). One of the isolated genes produced PHA copolymer when expressed in Pseudomonas putida. 78 Moreover, partial phaC genes were also obtained from metagenomic DNA via direct PCR 79 amplification (Foong et al. 2014; Pärnänen et al. 2015; Tai et al. 2015). Building on these previous 80 studies, we constructed a PHA- strain of P. putida KT2440 to use as a surrogate host and 81 functionally identified phaC genes after screening millions of agricultural wheat soil metagenomic 82 clones. A total of 27 PHA+ clones were obtained. Accumulation and monomer composition of PHA 83 directed by seven of these clones were further examined. 84 85 Methods 86 Bacterial strains, plasmids, cosmids and growth conditions 87 Bacterial strains, plasmids and cosmids are listed in Table 1. E. coli strains were grown at 88 37°C in LB (Lennox) medium (1% tryptone (w/v), 0.5% yeast extract (w/v), and 0.5% NaCl (w/v), 89 pH 7). Pseudomonas strains were grown at 30°C in LB or 0.1N M63 minimal medium (Escapa et al. 90 2011) supplemented with 0.5% sodium octanoate (w/v), 0.5% nonanoic acid (v/v) or 1% gluconic 91 acid (w/v). S. meliloti was grown in LB or YM medium (Schallmey et al. 2011). Antibiotics were 92 used at the following concentrations: streptomycin, 200 µg/ml for S. meliloti and 100 µg/ml for E. 93 coli; kanamycin, 100 µg/ml for Pseudomonas and 50 µg/ml for E. coli; neomycin, 200 µg/ml; 94 rifampicin, 100 µg/ml; gentamicin, 10 µg/ml for E. coli and 100 for P. putida; and tetracycline 20 95 µg/ml for E. coli or 40 µg/ml for P. putida. 96 97 Construction of PHA- strain PpUW2 98 DNA oligonucleotides are listed in Table 2. A RifR spontaneous mutant PpUW1 of P. putida 99 KT2440 was generated by plating a culture of strain KT2440 on a LB Rif plate, followed by single 5 bioRxiv preprint doi: https://doi.org/10.1101/042705; this version posted March 10, 2016. The copyright holder for this preprint (which was not certified by peer review) is the author/funder, who has granted bioRxiv a license to display the preprint in perpetuity. It is made available under aCC-BY 4.0 International license. 100 colony purification. To construct the PHA deficient strain PpUW2 (phaC1ZC2) of P. putida PpUW1, 101 a 943-bp DNA fragment containing 5´-phaC1 region (766 bp) was PCR amplified using P.
Details
-
File Typepdf
-
Upload Time-
-
Content LanguagesEnglish
-
Upload UserAnonymous/Not logged-in
-
File Pages48 Page
-
File Size-