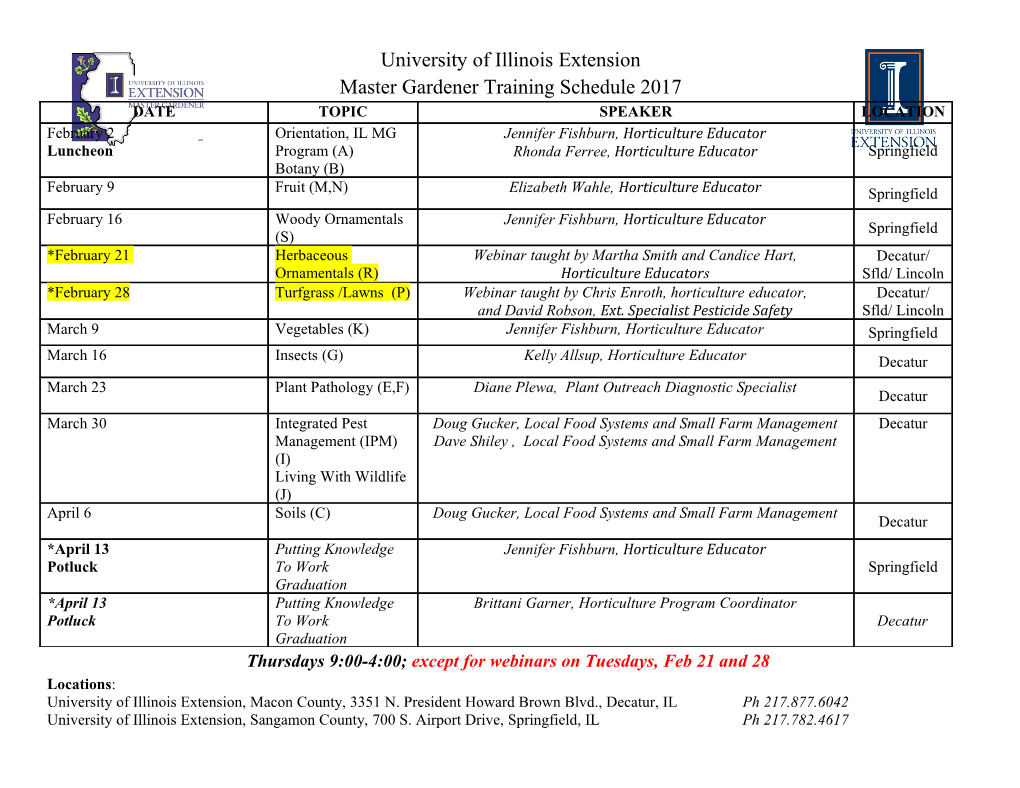
— 29 — Infrared imaging bolometers Harry A. C. EatonI Abstract Infrared imaging bolometers have found great utility in space imaging appli- cations. Bolometers measure incident energy by observing a temperature change caused when a material absorbs photons. Because there is great flexibility in choos- ing the absorbing material characteristics, bolometers can readily be designed to have narrow or broad-band characteristics over a wide range of wavelengths. Ima- ging bolometers are simply formed from arrays of individual bolometers in the focal plane of a telescope. Uncooled bolometers with high pixel counts are applied for studying the Sun and planets, while imaging bolometers cooled to very low tem- peratures but having only a few pixels have been designed for deep-space imaging applications, typically in the very-long wavelength to sub-millimetre region. Ultra- low temperature imaging bolometers have achieved detectivities better than the best photo-current detectors in the far-infrared region. Introduction Bolometers are capable of detecting radiant energy over a very broad spec- tral range but have found their greatest utility in the infrared range. Most recent developments of imaging bolometers have focused on the long-wave IR (LWIR) ñ range from 8 ñm to 13 m because the atmosphere is fairly transparent in this range, which also encompasses the peak emission from warm objects on Earth. While these developments are driven by commercial and military market interest the resulting technologies are quite usefully adapted to space applications. Many commercial imaging bolometers that are designed for the LWIR can be adapted ñ ñ ñ for use in short-wave (SWIR, 1 ñmto 3 m) and midwave (MWIR, 3 mto 8 m) ranges. With somewhat greater difficulty they can be adapted for far infrared or very broad-band applications as well (Foukal and Libonate 2001). For faint or cold astronomical targets, cryogenically cooled very long-wave IR (VLWIR) and sub- millimetre bolometer arrays have been developed, and these have recently been adapted for use in space. IJohns Hopkins University Applied Physics Laboratory, Laurel MD, USA 477 478 29. Infrared imaging bolometers History In the 1980s the Defense Advanced Research Project Agency (DARPA) funded researchers at Honeywell International and Texas Instruments to develop small un- cooled infrared imagers using micro-bolometer arrays. The results of this research were declassified in 1992 and have led to an explosive growth in both research on bolometer-based imagers as well as commercially available bolometer focal-plane arrays (FPA). The first uncooled micro-bolometer FPA was flown in space in 1997 (Spinhirne et al 2000). Since that time there have been many space missions incor- porating micro-bolometer FPAs, most of which have been for Earth and planetary observations. The first cryogenically cooled IR bolometer array to fly in space was the FIRP instrument on board the Japanese IRTS satellite flown in 1995 (Hirao et al 1996). Since then major advancements in low-temperature bolometers have resulted in detectivities surpassing those of photon-detector technologies. Bolometer fundamentals In its simplest form, a bolometer consists of an energy-absorbing material con- nected to a thermometer with a weak thermal connection to a constant-temperature heat sink. Incident radiant energy heats the absorber, raising its temperature, which is measured by the thermometer. A wide range of materials can be used as the energy absorber, allowing bolometer designers significant freedom to con- trol the operating spectral range, including broad and narrow tuning ranges in wavelength, λ. Figure 29.1 shows the schematic structure of a bolometer element. The absorber/thermometer’s heat capacity is Cp while Gc represents the equiva- lent thermal conductance from the element to the heat sink including (linearized approximations of) any radiative and convective components. Solving the heat- balance equation (ignoring any self-heating of the thermometer) gives the rise in the detector element temperature ε(λ)P (λ) ∆T = dλ , (29.1) 2 2 2 Z Gc + ω Cp q where ε is the emissivity of the absorber, P is the power incident on the absorber element, and ω is the frequency of the incident power envelope. Since low Gc im- proves the bolometer performance, steps are taken to minimize it including using low-emissivity materials on the detector back side (facing the heat sink), evacuated packages and long, thin support and connecting elements with low thermal con- 7 ductivity. Values for Gc of 10 W/K are typical for micro-bolometer arrays. The speed of response of a bolometer is governed by the bolometer time constant Cp τb = , (29.2) Gc and is much slower than most photon detectors. For micro-machined structures found in typical bolometer FPAs, the heat capacity is low enough for response times of the order of a few milliseconds. 479 Figure 29.1: A schematic representation of a bolometer element. Incident energy from above heats the absorber element. The maximum bolometer detectivity is limited by random fluctuations in the radiant power exchange between the detector and scene and is given by (Rogalski 2002) ε D∗ = , (29.3) b 8 k σ (T 5 + T 5) r B d s where kB is Boltzmann’s constant, σ is the Stefan-Boltzmann constant, Td is the detector temperature, and Ts is the scene temperature. This formula provides an upper bound for bolometer detectivity. In practice additional noise sources in the thermometer and phonon noise of the detector element reduce the detectivity. As can be seen from Equation 29.3, it is desirable that the detector be colder than the scene temperature, but there are diminishing returns for additional cooling. In practice this means that observations of warm targets such as the Sun, Earth and planets can easily be accomplished with detectors near room temperature, but astrophysical observations must been done with detectors cooled to a few kelvins or less. Uncooled bolometer arrays The primary figure of merit for uncooled FPA performance is the noise equiva- lent temperature difference (NETD, ∆TNE). NETD is the temperature difference across a black-body source that produces a signal difference equal to the rms de- tector noise. It is given by: 4 F 2 B ∆T = , (29.4) NE π ∗ ∂R A D ∂T r where F is the ratio of the focal length of the optics to its aperture diameter, ∂R ∂T is the derivative of the black-body radiance with respect to temperature at the wavelength of operation, D∗ is the actual pixel detectivity, B is the noise bandwidth 480 29. Infrared imaging bolometers of the measurement, and A is the area of the detector pixel. NETD is dependent on integration time (frame rate) because the detector noise is a function of the measurement bandwidth. The area dependence of the NETD makes the filling factor of the array an important parameter to optimize. NETDs as low as 23 mK ñ have been reported for micro-bolometer arrays having 50 ñm 50 m pixel size with F/1 optics operating at room temperature at 60 frames per× second (Howard 2000). For cryogenically cooled detectors the NETD is not reported because the temperature derivative of radiance is very small at cold temperatures. Thermometers Various technologies are available to measure the temperature rise of the de- tector element. These include temperature-dependent resistance (thermistors), py- roelectric and ferroelectric effects, thermopiles, and mechanical cantilevers. Each technique has various advantages and disadvantages, especially for FPAs. Tempe- rature-dependent resistive sensors are dominant in the market at present and have the advantages of monolithic array fabrication (with resulting low cost) and very good detectivity; drawbacks include complex element trimming circuitry and high 1/f noise. Pyroelectric and ferroelectric detectors sense changes in temperature and therefore require a chopper to modulate the signal, but are not affected by 1/f noise and are minimally affected by static offset variations across the array. Pyroelectric materials also require hybrid assembly techniques that raise the Gc and Cp values, but they do have the advantage that the hybrid structure can be coated with materials that are also not amenable to monolithic processing such as gold-black. Thermopiles (Kanno et al 1994) allow high linearity and negligible 1/f noise, but suffer from low signal levels with resulting poor detectivities and NEDT. Mechanical cantilever sensors operate at DC and theoretically have better noise performance (Datskos et al 2004), but require bulky and complex readout systems. Temperature-dependent resistance sensors (thermistors) Vanadium oxide (VOx) is the dominant temperature-sensitive resistance mate- rial used for uncooled monolithic IR FPAs, although many FPAs use amorphous silicon. A very large amount of research and technology development has gone into perfecting monolithic FPAs based on these technologies. Much of the improvements have focused on improving spatial NETD problems through on-array correction techniques. The growing trend towards higher pixel counts has resulted in smaller pixel sizes, yet reductions in circuit noise have kept pace to maintain similar NETD (Murphy 2002). Resistance-type micro-bolometer FPAs have been used in several Earth-observation and planetary space missions (Murphy et al 2000; Corlay and Arnolfo 2001; Lancaster et al 2003; Pope et al 2006). Pyroelectric sensors Among the uncooled IR FPAs the pyroelectric FPAs gained favour rapidly. This was primarily due to the superior uniformity and resulting good image qua- lity. Another advantage to such capacitive temperature sensors is that there is no 481 self-heating of the temperature sensing element. The main drawback to the techno- logy is the hybrid fabrication technique required to integrate the sensor into the electronic readout, even though this was actually an advantage for an application to solar astronomy because it allowed a relatively simple means to coat the detector with a thin film of gold-black to establish a broad, flat spectral response.
Details
-
File Typepdf
-
Upload Time-
-
Content LanguagesEnglish
-
Upload UserAnonymous/Not logged-in
-
File Pages7 Page
-
File Size-