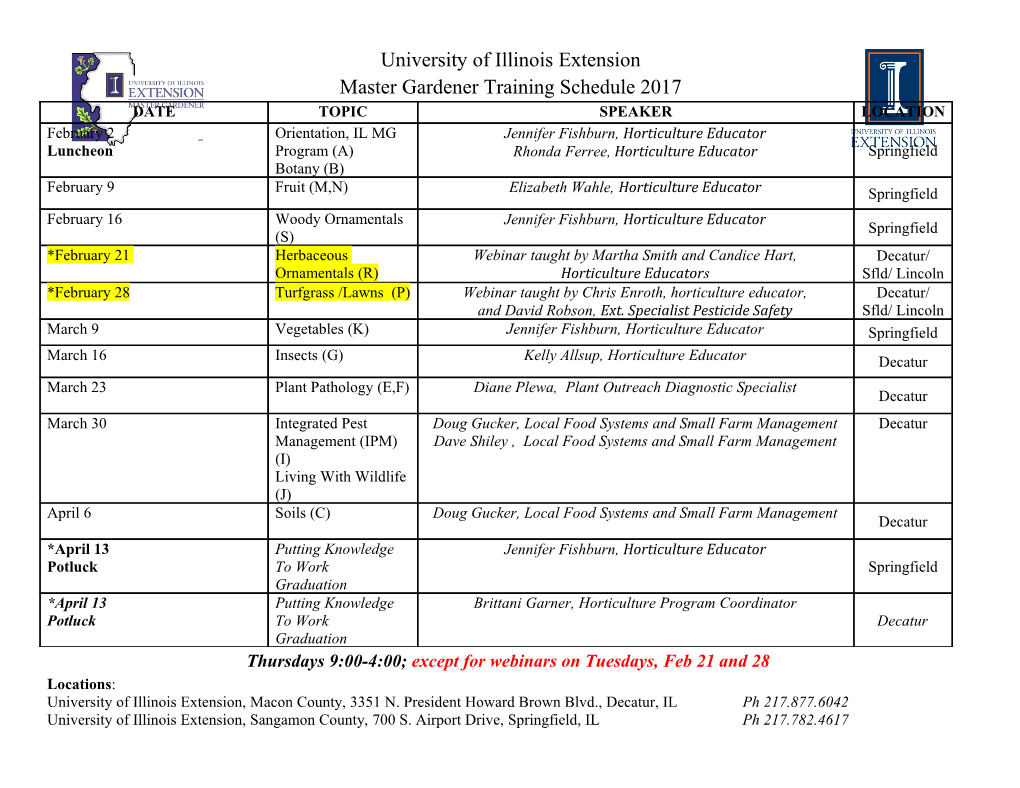
1 Revision 1 2 The high-pressure phase of lawsonite: A single crystal study of a key mantle hydrous phase 3 4 Earl O’Bannon III,1* Christine M. Beavers1,2, Martin Kunz2, and Quentin Williams1 5 1Department of Earth and Planetary Sciences, University of California, Santa Cruz, 1156 High 6 Street, Santa Cruz, California 95064, U.S.A. 7 2Advanced Light Source, Lawrence Berkeley National Laboratory, Berkeley, California, 94720, 8 U.S.A. 9 *Corresponding Author 10 1 11 Abstract 12 Lawsonite CaAl2Si2O7(OH)2·H2O is an important water carrier in subducting oceanic 13 crusts, and the primary hydrous phase in basalt at depths greater than ~80 km. We have 14 conducted high-pressure synchrotron single-crystal x-ray diffraction experiments on natural 15 lawsonite at room temperature up to ~10.0 GPa to study its high-pressure polymorphism. We 16 find that lawsonite remains orthorhombic with Cmcm symmetry up to ~9.3 GPa, and shows 17 nearly isotropic compression. Above ~9.3 GPa, lawsonite becomes monoclinic with P21/m 18 symmetry. Across the phase transition, the Ca polyhedron becomes markedly distorted, and 19 the average positions of the H2O molecules and hydroxyls change. The changes observed in the 20 H-atom positions under compression are different than the low temperature changes in this 21 material. We resolve for the first time the H-bonding configuration of the high-pressure 22 monoclinic phase of lawsonite. A bond valence approach is deployed to determine that the 23 phase transition from orthorhombic to monoclinic is primarily driven by the Si2O7 groups, and in 24 particular it's bridging oxygen atom (O1). The changes in the structure strongly indicate that 25 entropy increases across the symmetry-lowering transition, and hence that the slope of the 26 phase transition is negative. Therefore, monoclinic lawsonite is thus stable under the pressure 27 and temperature conditions that exist in the Earth, and is likely to be a major water carrier in 28 colder, deep subducted slabs. Monoclinic lawsonite also likely has enhanced electrical 29 conductivity along its c-axis due to the dynamically disordered hydrogen atoms. 30 Key words: Lawsonite; CaAl2Si2O7(OH)2·H2O; Single-crystal diffraction; High-Pressure; Hydrogen 31 disorder 32 1. Introduction 2 33 Significant amounts of water are transported into the mantle by subduction zones in the 34 form of hydrated minerals in oceanic crust and continentally derived meta-sediments [Ono, 35 1998; Chinnery et al., 2000; Dobrzhinetskaya and Green, 2007]. This water affects the chemical 36 and physical properties of materials by lowering their solidus temperatures, which allows for 37 partial melting of mantle material at relatively low temperatures, and by altering rheological 38 properties. Water reservoirs in subducted oceanic crust and its overlying sediments include 39 chloritoid, lawsonite, topaz, serpentines, chlorite (clinochlore), zoisite, amphiboles and micas. 40 Lawsonite is particularly interesting because of its high overall water content, ~11.5 wt.% [Baur, 41 1978], large stability field, and its prevalence in fully hydrated mid-ocean ridge basalt (MORB) 42 at pressure, which contains 7-15 wt% lawsonite [Schmidt and Poli, 1998]. Lawsonite and 43 phengitic mica are thought to be the dominant water carriers in subducted crust from ~200 to 44 over 300 km depth, which corresponds to pressures of ~7-10 GPa [Pawley, 1994; Schmidt and 45 Poli, 1994; Domanik and Holloway, 1996]. The way that H2O molecules and OH groups are 46 contained within the lawsonite structure is unusual (Figure 1). H2O molecules reside in cavities 47 that are formed by rings of two Si2O7 groups and two Al-octahedra. These cavities are not 48 completely open channels, like the channels typically found in ring silicates (e.g. cordierite), but 49 the channels in lawsonite also contain the large Ca ion, bound to the ring framework, in 50 addition to the water molecule. The OH groups are contained within a much smaller channel in 51 the structure formed by two AlO6 octahedra and two SiO4 tetrahedra. 3 52 53 Figure 1. Room pressure and temperature structure of lawsonite looking along the a-axis. Atom 54 colors are the same in all structural figures. All structural diagrams were generated using 55 CrystalMaker ® v.8.7.6. 56 Lawsonite undergoes two low temperature phase transitions at 155(5) and 273(5) K at 57 ambient pressure that involve changes in the hydrogen bonding of the H2O and OH groups 58 [Libowitzky and Armbruster, 1995], and to a lesser extent changes in the aluminosilicate 59 framework. However, no phase transitions are observed upon heating to ~600 oC [Pawley et al., 60 1996]. Notably, the H atoms of the H2O and OHs in lawsonite are dynamically disordered and 61 oscillate between two equivalent sites, and the low temperature phase transitions are 62 interpreted to be generated by a freezing in of the dynamic hopping motions of the H atoms 63 [Libowitzky and Armbruster, 1995; Libowitzky, 2009]. High pressure, room temperature single- 64 crystal diffraction structures have been reported up to ~3.78 GPa [Comodi and Zanazzi, 1996] 4 65 and no phase transitions were reported in this pressure range. An additional phase transition at 66 ~4 GPa from orthorhombic C to an orthorhombic P space group was reported by Boffa Ballaran 67 and Angel (2003) using single-crystal diffraction, but they could not determine the structure 68 and space group of this phase At higher pressures, Scott and Williams (1999) reported a 69 discontinuous shift in the O-H stretching vibrations at ~8-9 GPa in the high-pressure infrared 70 spectra of lawsonite. Daniel et al. (2000) reported a phase transition at ~8.6 GPa using Raman 71 spectroscopy and powder diffraction, and Pawley and Allan (2001) observed the same 72 transition at ~10-11 GPa using powder diffraction. This transition, from orthorhombic to 73 monoclinic symmetry, has now been known, for ~16 years, to occur between ~8.6 and ~11 GPa 74 [Daniel et al., 2000; Pawley and Allan, 2001; Boffa Ballaran and Angel, 2003]. 75 Structural determinations of the proposed orthorhombic P phase (> 4 GPa) and the 76 monoclinic P phase have not been reported, and both of these transitions occur at pressures 77 relevant to subduction zonesPawley and Allan (2001) reported a Rietveld refinement of the 78 P21/m structure of monoclinic lawsonite using the orthorhombic Cmcm structure as their 79 starting model. The P-T slope of this phase transition is poorly constrained, and Pawley and 80 Allan (2001) hypothesize that this transition has a shallow positive slope. If this were the case, 81 the high-pressure phase of lawsonite would be unlikely to be stable under conditions found 82 within subduction zones. However, Pawley and Allan (2001) also explicitly state that a negative 83 slope cannot be ruled out, which would intersect the temperature field within colder subducted 84 slabs. Moreover, the high-pressure phase of lawsonite almost certainly has higher entropy since 85 the oxygen position, O2, splits into two separate sites in the monoclinic structure , O2A and 86 O2B, and the cation site M1 splits into M1A and M1B (e.g. Liebscher et al., 2010; Pawley and 5 87 Allan, 2001). Hence, ΔSortho-mono is anticipated to be positive, and ΔVortho-mono is small but 88 negative (e.g. Boffa Ballaran and Angel, 2003; Daniel et al., 2000; Pawley and Allan, 2001), 89 which indicates that the slope of the Cmcm-P21/m transition must be negative. From this it 90 follows that the high-pressure monoclinic phase of lawsonite could be the dominant water 91 carrier in basalts carried by rapidly subducted, older slabs, and thus may be of considerable 92 importance for moving water to depths deeper than 300 km (~10 GPa), and approaching the 93 top of the transition zone. 94 High quality single-crystal structural determinations for the high-pressure phase(s) of 95 the dominant carrier of water to depths of ~300 km in subducted basalt [Schmidt and Poli, 96 1998] and in hydrated metamorphic assemblages associated with subduction [Vitale Brovarone 97 and Beyssac, 2014] have thus not yet been reported, and the structure of this primary water 98 carrier is uncertain. This study uses synchrotron based single-crystal x-ray diffraction at room 99 temperature to examine the structural changes of lawsonite under compression up to ~10.0 100 GPa. The great benefit of single-crystal structure determinations is the ability to ab initio 101 determine, and subsequently refine the atomic positions. Moreover, atomic positions can be 102 refined with anisotropic displacement parameters (ADPs), which is often not possible based on 103 the reduced data quality obtained by high pressure poeder diffraction.. Here, our goals are to 104 probe and characterize the existence of the proposed ~4.0 GPa transition in lawsonite, and 105 solve the structure of the higher-pressure monoclinic lawsonite. Both the proposed 106 orthorhombic P phase and the monoclinic P phase occur within pressure ranges that are 107 important for our understanding of subduction zones, and both are thus anticipated to be 108 primary carriers of water to depth. 6 109 2. Methods 110 2.1 Sample characterization 111 The natural sample used in this study is from the type locality Reed Station, Tiburon 112 Peninsula, Marin Co., California, USA [Ransome, 1895]. We characterized the sample with 113 single-crystal diffraction and Raman spectroscopy, which both agree well with previous studies 114 [Baur, 1978; Daniel et al., 2000; Meyer et al., 2001; Pawley and Allan, 2001]. Ambient pressure 115 measurements were conducted on Beamline 11.3.1 at the Advanced Light Source (ALS) at 116 Lawrence Berkeley National Lab in Berkeley, CA.
Details
-
File Typepdf
-
Upload Time-
-
Content LanguagesEnglish
-
Upload UserAnonymous/Not logged-in
-
File Pages30 Page
-
File Size-