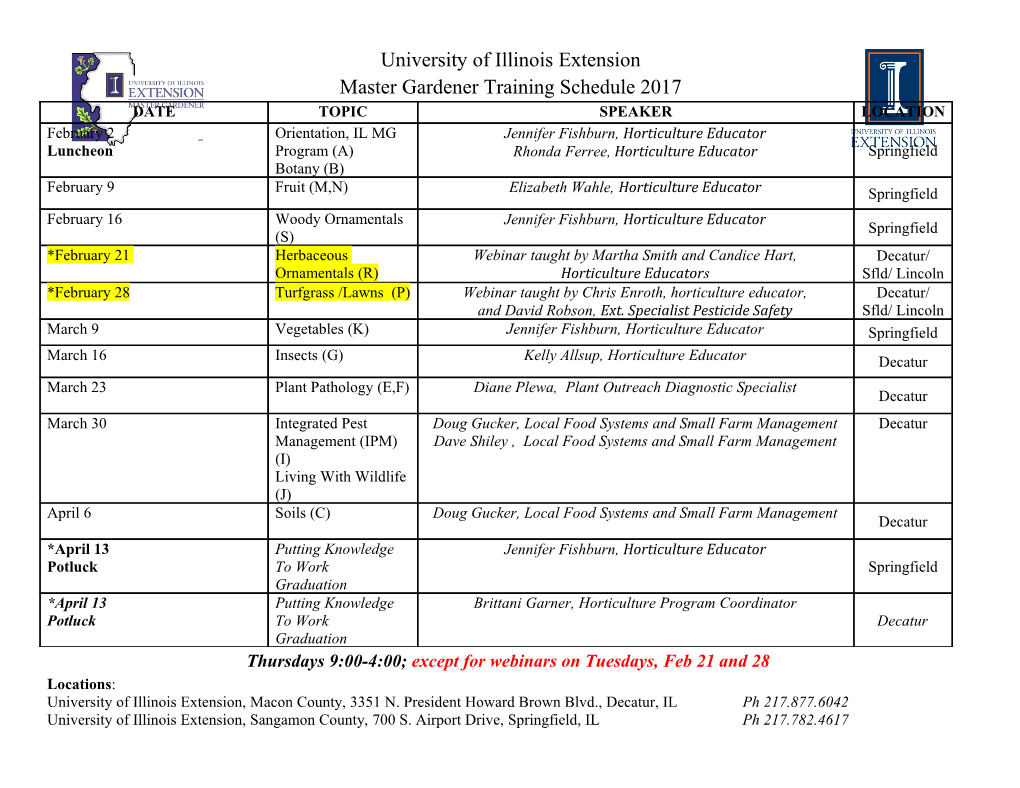
BIOMEDICAL APPLICATIONS OF MEMS Jack W. Judy University of California, Los Angeles 68-121 Engineering IV, Box 159410 Los Angeles, CA 90095-1594 Tel: (310) 206-1371 [email protected] Abstract – Micromachining and MEMS For a discussion of the early work in MEMS, technologies can be used to produce complex including many of the seminal papers, the interested electrical, mechanical, fluidic, thermal, optical, reader is directed to reference [1]. For a and magnetic structures, devices, and systems comprehensive discussion of micromachining on a scale ranging from organs to subcellular processes and MEMS devices, the interested reader organelles. This miniaturization ability has is directed to the texts by Kovacs [2] and Madou [3]. enabled MEMS to be applied in many areas of biology, medicine, and biomedical engineering – MICROFABRICATION a field generally referred to as BioMEMS. The future looks bright for BioMEMS to realize (1) Although many of the microfabrication techniques microsensor arrays that act as an electronic and materials used to produce MEMS have been nose or tongue, (2) microfabricated neural borrowed from the IC industry, the field of MEMS has systems capable of controlling motor or sensory also driven the development and refinement of other prosthetic devices, (3) painless microsurgical microfabrication processes and non-traditional tools, and (4) complete microfluidic systems for materials. total chemical or genetic analyses. Conventional IC Processes and Materials: INTRODUCTION - photolithography; thermal oxidation; dopant diffusion; ion implantation; LPCVD; PECVD; Microelectromechanical systems (MEMS) is a evaporation; sputtering; wet etching; plasma technology of miniaturization that has been largely etching; reactive-ion etching; ion milling adopted from the integrated circuit (IC) industry and applied to the miniaturization of all systems (i.e., not - silicon; silicon dioxide; silicon nitride; aluminum only electrical systems but also mechanical, optical, fluidic, magnetic, etc). Miniaturization is Additional Processes and Materials used in MEMS: accomplished with microfabrication processes, such - anisotropic wet etching of single-crystal silicon; as micromachining, that typically use lithography, deep reactive-ion etching or DRIE; x-ray although other non-lithographic precision lithography; electroplating; low-stress LPCVD microfabrication techniques exist (FIB, EDM, laser films; thick-film resist (SU-8); spin casting; machining). Due to the enormous breadth and micromolding; batch microassembly diversity of the field of MEMS, the acronym is not a particularly apt one. However, it is used almost - piezoelectric films such as PZT; magnetic films universally to refer to the entire field (i.e., all devices such as Ni, Fe, Co, and rare earth alloys; high produced by micromachining). Other names for this temperature materials such as SiC and ceramics; general field include “microsystems”, popular in mechanically robust aluminum alloys; stainless Europe, and “micromachines”, popular in Asia. steel; platinum; gold; sheet glass; plastics such as PVC and PDMS The methods used to integrate multiple patterned typically the case for surface micromachining). materials together to fabricate a completed MEMS Exploiting the predictable anisotropic etching device are just as important as the individual characteristics of single-crystal silicon, many high- processes and materials themselves. The two most precision complex 3-D shapes, such as V-grooves, general methods of MEMS integration are described channels, pyramidal pits, membranes, vias, and in the next two sub-sections: surface micromachining nozzles, can be formed [5]. An illustration of a typical and bulk micromachining. bulk micromachining process is given in Figure 2. Surface Micromachining (100) Silicon Wafer Simply stated, surface micromachining is a method p+ Silicon of producing MEMS by depositing, patterning, and etching a sequence of thin films, typically ~1 µm Silicon Nitride Film thick. One of the most important processing steps that is required of dynamic MEMS devices is the selective removal of an underlying film, referred to as a sacrificial layer, without attacking an overlaying film, referred to as the structural layer, used to create the mechanical parts. Figure 1 illustrates a typical {111 Planes} surface micromachining process [4]. Surface micromachining has been used to produce a wide variety of MEMS devices for many different applications. In fact, some of them are produced commercially in large volumes (> million parts per month). Through Membrane V-Groove Hole Anchor Sacrificial Layer Substrate Figure 2: Bulk micromachining. Structural Layer Substrate Bonding Silicon, glass, metal and polymeric substrates can be bonded together through a variety of processes (i.e., fusion bonding, anodic bonding, eutectic bonding, and adhesive bonding). Typically at least one of the Release Etch bonded substrates has been previously micromachined. Substrate bonding is typically done to achieve a structure that is difficult to form otherwise (i.e., large cavities that may be hermetically sealed or a complex system of enclosed channels) or simply to add mechanical support and protection [2]. Figure 1: Surface micromachining and the sacrificial layer technique. Non-Silicon Microfabrication Bulk Micromachining The development of MEMS has contributed significantly to the improvement of non-silicon Bulk micromachining differs from surface microfabrication techniques. Two prominent micromachining in that the substrate material, which examples are LIGA and plastic molding from is typically single-crystal silicon, is patterned and micromachined substrates. shaped to form an important functional component of the resulting device (i.e., the silicon substrate does not simply act as a rigid mechanical base as is LIGA comprehensive discussion of all the different sensors and their applications is beyond the scope of this LIGA is a German acronym standing for lithographie, paper. Instead, a discussion of several classes of galvanoformung (plating), and abformung (molding) microsensors and an overview of their applications [6]. However, in practice LIGA essentially stands for will be given. a process that combines extremely thick-film resists (often >1 mm) and x-ray lithography, which can Microsensors for Biomechanics pattern thick resists with high fidelity and results in vertical sidewalls. Although some applications may Studies of the forces created by and imposed on the require only the tall patterned resist structures body benefit from increasing the sensitivity of themselves, other applications benefit from using the mechanical stress and strain sensors while also thick resist structures as plating molds (i.e., material reducing their size and cost. The following are can be quickly deposited into the mold by examples of microsensors used to study electroplating). A drawback to LIGA is the need for biomechanics. high-energy x-ray sources (e.g., synchrotrons or linear accelerators) that are very expensive and rare Strain Gauges (i.e., only several such sources exist in the U.S.). Strain gauges are used to characterize the forces in SU-8 the body. Since silicon is known to be an excellent piezoresistive material (i.e., it’s resistance changes Recently a cheap alternative to LIGA, with nearly the as a function of applied force), it can be easily same performance, has been developed. The micromachined to form sub-millimeter multi-axis solution is to use a special epoxy-resin-based optical strain gauges [10]. Applications of such miniaturized resist, called SU-8, that can be spun on in thick strain gauges include orthopedic research and the layers (>500 µm), patterned with commonly available study of muscles. Although the understanding of contract lithography tools, and yet still achieves muscle function and structure is well understood at vertical sidewalls [7]. the whole-muscle and cellular levels, muscles have not been well characterized in the region in between. Plastic Molding with PDMS An improved understanding at this level would allow for the development of improved locomotion Polydimethylsiloxane (PDMS) is a transparent therapies and prosthetic devices. elastomer that can be poured over a mold (e.g., a wafer with a pattern of tall SU-8 structures), Accelerometers polymerized, and then removed simply by peeling it off of the mold substrate [8]. The advantages of this One class of microsensors that MEMS technology process include (1) many inexpensive PDMS parts has had the most positive impact on are inertial can be fabricated from a single mold, (2) the PDMS sensors (i.e., accelerometers and gyros). Since will faithfully reproduce even sub-micron features in inertial devices typically consist of a proof mass, the mold, (3) PDMS is biocompatible and thus can mechanical flexure, and displacement sensor, be used in a variety of BioMEMS applications, and MEMS technology is well suited to integrate each of (4) since PDMS is transparent, tissues, cells, and these sensor elements into a single chip. In fact, it is other materials can be easily imaged through it. also possible to integrate ICs with the micro- Common uses of PDMS in biomedical applications mechanical elements to add signal amplification and include: microstamping of biological compounds (to filtration capability to the chip-scale sensor [11]. observe geometric behavior of cells and tissues) and Inertial microsensors are useful to determine impact microfluidics systems [9][8]. level and patient posture. BIOMEDICAL
Details
-
File Typepdf
-
Upload Time-
-
Content LanguagesEnglish
-
Upload UserAnonymous/Not logged-in
-
File Pages12 Page
-
File Size-