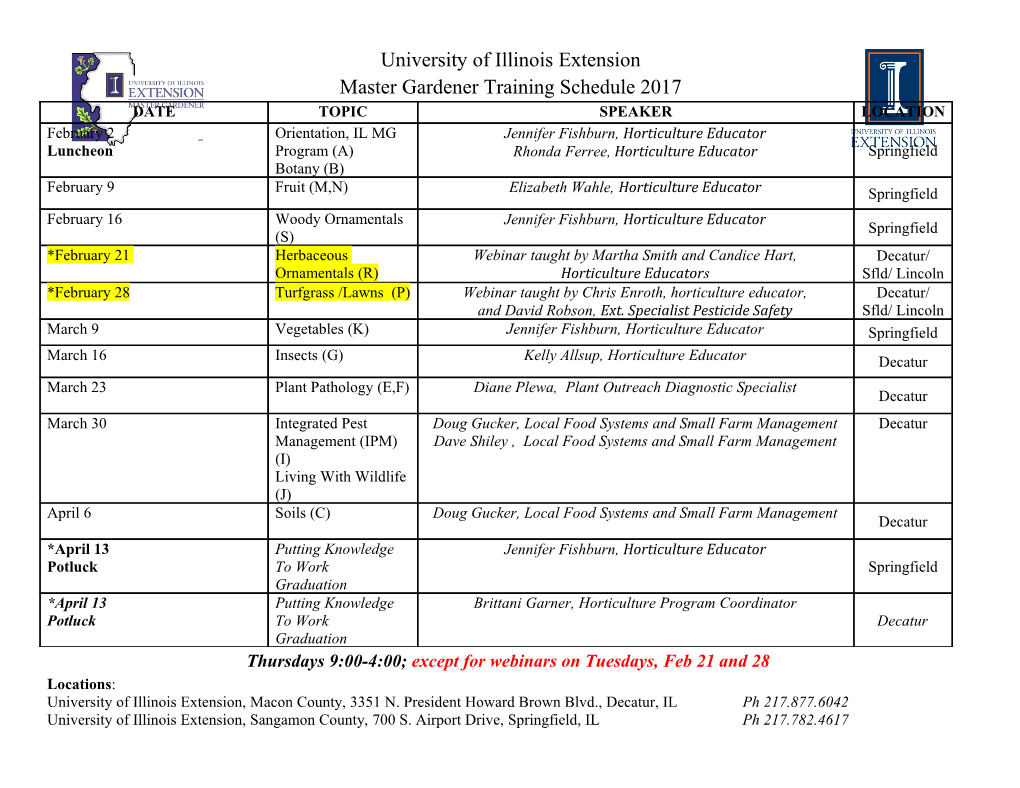
MIAMI UNIVERSITY The Graduate School Certificate for Approving the Dissertation We hereby approve the Dissertation of Zhenxin Hu Candidate for the Degree: Doctor of Philosophy _______________________________________________ Director Dr. Michael Crowder _______________________________________________ Reader Dr. Chris Makaroff _______________________________________________ Reader Dr. Michael Kennedy _______________________________________________ Reader Dr. John Hawes _______________________________________________ Graduate School Representative Dr. Eileen Bridge ABSTRACT KINETIC AND SPECTROSCOPIC STUDIES OF L1, THE METALLO-β- LACTAMASE FROM Stenotrophomonas maltophilia by Zhenxin Hu Metallo-β-lactamase L1 (mβl L1), originally from Stenotrophomonas maltophilia, can hydrolyze all β-lactam containing antibiotics. Previous crystal structures showed that mβl L1binds two Zn(II) ions in the active site, and there is a long flexible loop above the metal center. To better understand the function of the Zn(II) ions in mβl L1, several metal-substituted and heterobimetallic analogs of L1 were generated and characterized using spectroscopic and kinetic studies. The metal binding sites in L1 can accommodate a number of different metal ions to afford catalytically-active analogs. Pre-steady state kinetic studies using nitrocefin as substrate showed that both Zn(II) ions were required for the formation of intermediate and that an analog of L1 containing only one equivalent of Zn(II) is slightly active. Different metal ions in the Zn2 metal binding site modulated mβl L1’s substrate preference, and L1 analogs containing Ni(II) or Fe in the Zn2 site were unable to hydrolyze penicillins but could hydrolyze cephalosporins and carbapenems. Rapid freeze quench (RFQ) EPR and 1H NMR spectra confirmed the catalytic function of both metal sites. Based on the kinetic and spectroscopic studies, a reaction mechanism of mβl L1 was proposed when nitrocefin is the substrate. In an effort to understand the function of the loop above the active site, fluorescence resonance energy transfer (FRET) and double electron electron resonance (DEER) studies were proposed. The preparation of double FRET labeled L1 analogs was unsuccessful; however, a double spin-labeled analog was made. Preliminary RFQ-DEER data show that this technique can be used to probe intramolecular motions during catalysis. The data from this dissertation can be used to guide future rational inhibitor design efforts. KINETIC AND SPECTROSCOPIC STUDIES OF L1, THE METALLO-β- LACTAMASE FROM Stenotrophomonas maltophilia A DISSERTATION Submitted to the Faculty of Miami University in partial fulfillment of the requirements for the degree of Doctor of Philosophy Department of Chemistry and Biochemistry by Zhenxin Hu Miami University Oxford, Ohio 2008 Dissertation Director: Dr. Michael Crowder TABLE OF CONTENTS Chapter 1: Introduction 1. β-Lactam containing antibiotics and β-lactam resistance 2 1.1 Discovery of penicillin 2 1.2 Different generations of penicillins 1.3 The outbreak of β-lactam resistance 5 1.4 General mechanisms for antibiotic resistance 5 2. Classification of β-lactamases 5 2.1. Ambler scheme 5 2.2 Metallo-β-lactamases 8 2.3 Previous studies on metallo-β-lactamases 8 2.3.1 X-ray crystallographic studies 8 2.3.2 Kinetic studies 11 2.3.3 Spectroscopic studies 12 2.3.4 Computational studies 12 3. Metallo-β-lactamase L1(Mβl L1) 13 3.1 Discovery of a metallo-β-lactamase in Xanthomonas (Pseudomonas) maltophilia. 13 3.2 Recombinant Mβl L1 13 3.3 Metal content of Mβl L1 13 3.4 Kinetic studies of Mβl L1 14 3.5 Crystal structure of Mβl L1 15 3.6 Mutational studies on Mβl L1 16 3.7 Metal substitution and spectroscopic studies on Mβl L1 20 4. Introduction to dissertation 21 5. References 23 ii Chapter 2: Folding strategy to prepare Co(II)-substituted metallo-β-lactamase L1 2.1 Introduction 32 2.2 Materials and Methods 34 2.3 Results 37 2.4 Discussion 47 2.5 Acknowledgement 49 2.6 References 50 Chapter 3: Metal content of metallo-β-lactamase L1 is determined by the bioavailability of metal ions 3.1 Introduction 59 3.2 Materials and Methods 61 3.3 Results 63 3.4 Discussion 73 3.5 References 76 Chapter 4: Role of the Zn1 and Zn2 sites in metallo-β-lactamase L1 4.1 Introduction 85 4.2 Materials and Methods 86 4.3 Results 90 4.4 Discussion 109 4.5 Acknowledgement 115 4.6 References 116 Chapter 5: Structure and mechanism of Cu- and Ni-substituted analogs of metallo-β- lactamase L1 5.1 Introduction 124 5.2 Materials and Methods 127 5.3 Results 128 5.4 Discussion 139 iii 5.5 References 143 Chapter 6: Probing the function of the flexible loop in metallo-β-lactamase L1 6.1 Introduction 150 6.2 Materials and Methods 153 6.3 Results 161 6.4 Discussion 172 6.5 Acknowledgement 180 6.6 References 181 Chapter 7 Conclusions 185 iv LIST OF TABLES Table 1.1: Classification scheme for metallo-β-lactamases. 9 Table 2.1: Characterization of Co(II)-substituted L1 analogs. 41 Table 2.2: Metal content and steady-state kinetic constants of Zn(II)-containing L1 analogs. 45 Table 3.1: Steady-state kinetic and metal content data for FL-L1. 66 Table 3.2: Steady-state kinetic and metal content data for L1 folded in the cytoplasm (M-L1). 68 Table 3.3: Characterization of L1 refolded in the presence of Fe(II), Zn(II), and Mn(II). 70 Table 4.1: Steady state kinetic parameters and metal content of HXXC mutants of L1. 91 Table 4.2: Steady state kinetics of different metal bound analogs of L1. 93 Table 4.3: Exponential fits to the stopped-flow kinetic data and kinetic simulations. 102 Table 5.1: Steady-state kinetic studies and metal analyses on Cu- and Ni-containing analogs of L1. 131 Table 5.2: Steady state kinetics of different L1 analogs with different substrates. 132 Table 6.1: Steady-state kinetics and metal analyses of L1 mutants. 163 Table 6.2: Steady state kinetic data with spin-labeled analogs of L1. 167 Table 6.3: Possible dipolar couplings in the T163/T265 double mutant of tetrameric L1. 178 v LIST OF FIGURES Figure 1.1: Representative β-lactam containing antibiotics. 3-4 Figure 1.2: Nitrocefin hydrolysis by β-lactamase. 6 Figure 1.3: Inhibition of serine β-lactamase by clavulanic acid. 7 Figure 1.4: Crystal structures of different metallo-β-lactamases. 10 Figure 1.5: The active site of Mβl L1 with hydrolyzed moxalactam. 17 Figure 1.6: Function of Asp120 in the active site of L1. 18 Figure 2.1: Active site of L1. 33 Figure 2.2: UV-Vis spectra of cobalt-containing analogs of L1. 38 Figure 2.3: Fluorescence emission spectra of L1 samples. 43 Figure 2.4: Stopped-flow kinetic studies of Co(II)-substituted L1. 46 Figure 3.1: Localization of L1 produced in cells that contain the L1 gene and the leader sequence (FL-L1) and the L1 gene without the leader sequence (M-L1). 65 Figure 3.2: EPR of of Fe-containing L1. 71 Figure 4.1: UV-Vis and NMR spectra of 1Co-L1. 95 Figure 4.2: EPR spectra from metal-containing species of L1. 97 Figure 4.3: Stopped-flow traces of reaction of Zn(II)-containing L1 analogs and nitrocefin. 98 Figure 4.4: Stopped-flow traces of the reaction of Co(II)-containing L1 analogs with nitrocefin. 100 Figure 4.5: Intermediate formation by L1 analogs. 103 Figure 4.6: RFQ-EPR of ZnCo-L1 with nitrocefin. 104 Figure 4.7: Stopped-flow traces of Fe-containing L1 analogs reacted with nitrocefin. 107 Figure 4.8: RFQ-EPR of ZnFe-L1 with nitrocefin. 108 Figure 4.9: Proposed reaction mechanism of L1 for the hydrolysis of nitrocefin. 114 Figure 5.1: Structures of β-lactam antibiotics used as substrates in these studies. 125 Figure 5.2: Active site of Mβl L1. 126 Figure 5.3: The EPR spectrum of 670 μM Cu-L1. 133 Figure 5.4: 1H NMR spectra of Ni-L1. 135 Figure 5.5. Stopped-flow kinetic studies on Cu-L1. 136 vi Figure 5.6: Stopped-flow kinetic studies on Ni-containing L1. 138 Figure 6.1: Crystal structure of Mβl L1. 152 Figure 6.2: Structures of Cy3 (top) and Cy5 (bottom). 154 Figure 6.3: Scheme of spin labeling reaction of MTSSL and protein. 155 Figure 6.4: Scheme of four pulse DEER. 160 Figure 6.5: Fluorescence spectra of mutants. 164 Figure 6.6: MALDI-TOF MS of double spin labeled T163C/T265C. 167 Figure 6.7: EPR spectrum of (A) free MTSSL and (B) MTSSL-labeled T163C/T265C. 169 Figure 6.8: DEER spectra of resting double spin-labeled L1 mutant. 170 Figure 6.9: DEER spectra of T163C/T265C rapid freeze quenched with nitrocefin. 171 Figure 6.10: Sites on L1 where labels were attached. 174 Figure 6.11: Thr163 in tetrameric L1. 176 Figure 6.12: Structure of spin-labeled penicillin (SLPEN). 180 Figure 7.1: (Left) Carboxylate group (in red) in β-lactam containing compounds. (Right) 17O-labeled substrate. 189 Figure 7.2: Chimeric tetramer of L1. 190 Figure 7.3: 15N-labeled substrate. 191 Figure 7.4: Thiono analog of substrate. 191 Figure 7.5: Potential inhibitor for mβl L1. 192 Figure 7.6: (Left) Structure of first compound tested. (Right) IC50 plot of first compound reacted with L1 193 Figure 7.7: (Left) Structure of second compound tested. (Right) IC50 plot of second compound reacted with L1. 193 vii Acknowledgements I would like to sincerely thank my advisor, Dr. Crowder. It is my honor and privilege to do research under his guidance. His continuous encouragement, assistance, and support are indispensable for the completion of this effort.
Details
-
File Typepdf
-
Upload Time-
-
Content LanguagesEnglish
-
Upload UserAnonymous/Not logged-in
-
File Pages206 Page
-
File Size-