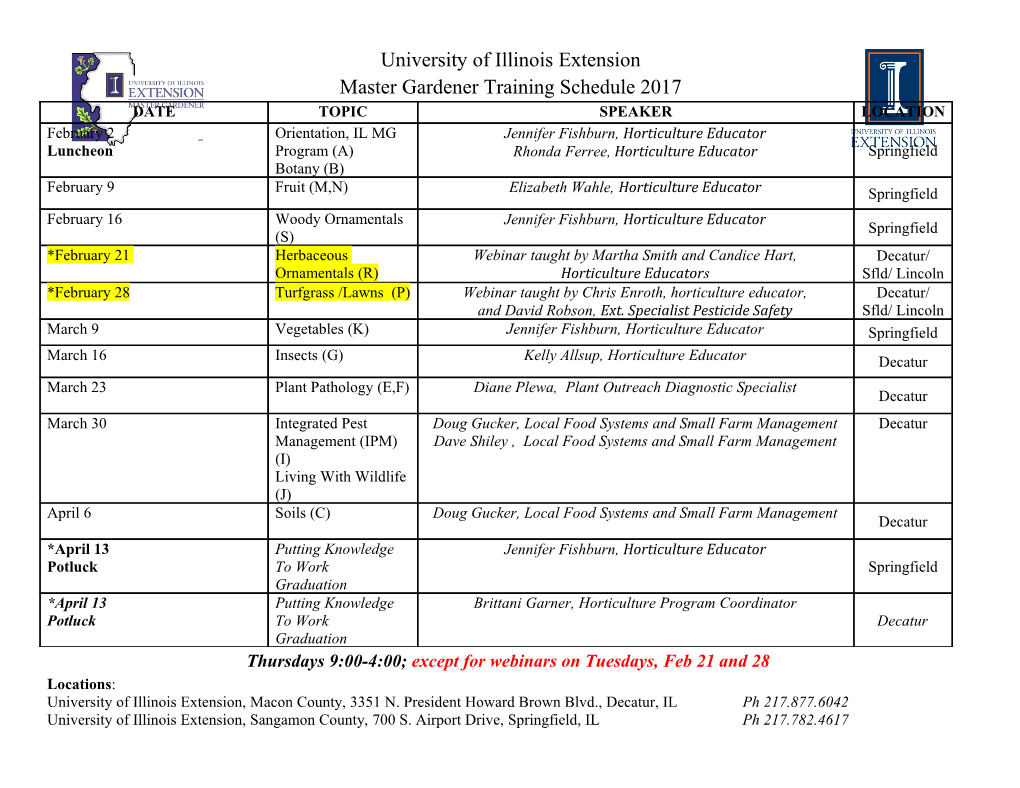
Review: Biochemistry of carbohydrate blood group antigens L.G. G ILLIVER AND S.M. H ENRY This review presents the basics of the structural glycan (the largest size expected for a carbohydrate chemistry of blood group glycoconjugates, with special epitope). This is compared with only 46,656 reference to red cell serology. Its aim is to create an combinations possible with a chain made up of six appreciation of the inherent subtleties of the amino acids, more than seven orders of magnitude carbohydrate blood group antigens, which are lower than the diversity possible in glycans. 1 currently poorly understood within the field of blood Fortunately, despite the vast potential for complexity in transfusion. It is hoped that a better understanding of carbohydrate chains, there is generally only a limited the intricacies of the carbohydrate blood group range of structures representing the carbohydrate systems will lead to further contributions to the body blood group epitopes (glycotopes), although many of of knowledge within this growing field. the structural differences are subtle and some epitope surfaces may be common. These subtleties may represent different blood group antigens and in some Introduction In 1900, Karl Landsteiner discovered the cases, specific antibodies may recognize the common carbohydrate blood group system of ABO after mixing surfaces. For example, anti-A,B recognizes the the cells and serum of his colleagues. Over the next similarities between A and B antigens, not the subtleties 100 years many other blood group systems were which distinguish them. 2 Unfortunately, this potential discovered and later resolved at both the molecular and variation makes structural determination of carbo- the genetic level. It is now clearly recognized that most hydrate antigens complex, requiring combinations of blood group systems are either carbohydrate, protein, powerful techniques such as nuclear magnetic or protein-carbohydrate in nature, although they may resonance spectroscopy and mass spectrometry to associate with other components and complexes to be elucidate the structures of carbohydrate antigens recognized as specific blood group determinants. without ambiguity. 3,4 Progress in determining the Essentially, a blood group antigen is any polymorphic structures of carbohydrate blood group antigens has three-dimensional conformation expressed on the been slow, hindered by a combination of factors, such outer membrane of a RBC against which an antibody is as the fact that carbohydrate blood group antigens available. The concept of the three-dimensional shape are not the primary product of a gene—being rather is very important because this is what antibodies secondary biosynthetic products—and that the recognize, and the number of potential shapes that can isolation and purification of carbohydrate blood group be found in carbohydrate chains is enormous. The antigens in requisite quantities is difficult. As a result, variability in characteristics such as sugar type, linkage we know only the basic structures of the major type, branch positioning, shape, charge, ring size, and glycotopes and antigens expressed in the carbohydrate epimeric (pairs of monosaccharides which differ only blood group systems. We have not yet characterized in the configuration about one carbon atom are many of the phenotypic subtleties, such as those that epimers of each other e.g., D-glucose and D-mannose determine ABO subgroups. are epimers with respect to the C2 carbon atom) and Because carbohydrate blood group antigens are 12 anomeric configuration results in more than 1.05 × 10 important to transfusion medicine and are becoming possible structural combinations from a six -sugar more recognized in transplantation (especially xeno- IMMUNOHEMATOLOGY, VOLUME 19, NUMBER 2, 2003 33 L.G. G ILLIVER AND S.M. H ENRY 5 transplantation ) and studies of micoorganism binding Carbon numbering D-glucose L-glucose and interactions, 6 it is becoming more important for serologists to understand the biochemistry of carbohydrate blood group antigens. We presently recognize several blood group systems and collections 7 where the blood group glycotopes are comprised entirely of carbohydrate, such as ABO, Lewis, H, Ii, P, Sd, Cad, etc.; or others, such as MN, where carbohydrate moieties are essential to the structure of the epitope; or even others, where carbohydrates are associated with the character of the antigen—e.g., GPI-linked membrane proteins such as Cromer, Dombrock, Cartwright, and John Milton Hagen, etc. Fig. 1. Carbon numbering of linear hexose sugars and the D- and L- This review is not intended to cover the structures of glucose. The carbon numbering of a generic 6-car - bon sugar is shown on the left (with the chiral center hydro - carbohydrate blood group systems, rather it is intended gens and hydroxyl groups not shown). Carbons 2, 3, 4, and 5 to provide a basic understanding of carbohydrate are the chiral centers of 6-carbon sugars. The enantiomeric form is designated by the direction of the hydroxyl group on blood group chemistry, and in this regard we will use the highest numbered chiral carbon (arrows)—in the case of ABO antigens as examples. 6-carbon sugars, this is carbon 5. In D-glucose (middle), the hydroxyl group of carbon 5 points to the right, and in L-glucose (right), this hydroxyl group points to the left. Monosaccharides The common blood group–related sugars, namely total number of carbons in the structure). If this hydroxyl group points to the right when the carbonyl -D-glucose (Glc), N -acetyl - -D-glucosamine (GlcNAc), β β group is at the top, the monosaccharide is said to be in β-D-galactose (Gal), N -acetyl -β-D-galactosamine the D configuration. The mirror image of this structure, (GalNAc), α-L-fucose (Fuc), and D-mannose (Man) are all six -carbon sugars (hexoses). The carbonyl group with the hydroxyl group pointing to the left, is the L (C=O) of each residue is an aldehyde (HC=O), and thus configuration. The D and L configurations of glucose they are all classified as aldohexoses. Another special are shown as open chain Fischer projection formulas monosaccharide which contains nine carbons is also (Fig. 1). In blood group glycoconjugates, sugars exist common in blood group antigens, with the most almost exclusively in the D configuration, except common blood group variation being N -acetyl - fucose, which is found in the L configuration. The chiral centers of saccharides can also be designated as α-D-neuraminic acid (also referred to as NeuAc or sialic acid). There are various forms of sialic acid, 8,9 but these R or S under the Cahn-Ingold-Prelog system. This is a will not be reviewed here. more modern notation; however, it is not often used in Sugar molecules can have configurational isomers carbohydrate chemistry because it causes confusion in which differ in their arrangement in space even though the classification of some monosaccharides. they have identical composition. The variation in the The seven common blood group sugars almost configuration of individual carbohydrates was first exclusively form pyran rings—six atoms forming the characterized in relation to the smallest chiral ring, compared with furan, which is a five-atom ring carbohydrate, glyceraldehyde, by German chemist Emil (Fig. 2). Fischer in the late 19th century. In extremely simple Numbering of the carbons in the pyranose ring terms, chirality is “handedness”—that is, the existence formation of hexose and nonose sugars is shown in of left/right apposition. For example, your left hand Figure 3. and right hand are mirror images and therefore“chiral.” The pyran ring comes about through the formation The enantiomeric (mirror image) configurations of an oxygen bridge between a carbon (carbon 1 in the Fischer described were designated D and L by Rosanoff common hexose blood group sugars, or carbon 2 in in 1906. 10 Today, all monosaccharides are classified sialic acid) and the oxygen of the hydroxyl group five according to this principle, which depends on the carbons away. 10 In hexoses, carbon 5 rotates so that its orientation of the hydroxyl group attached to the hydroxyl group apposes carbon 1. The double-bonded highest numbered chiral carbon (C (n -1) , where n is the oxygen on carbon 1 is reduced—taking the hydrogen 34 IMMUNOHEMATOLOGY, VOLUME 19, NUMBER 2, 2003 Review: carbohydrate blood group antigens 1 Generic furan ring Generic pyran ring D-galactose 2 β-D-galactofuranose Open chain β-D-galactopyranose D-galactose 3 Fig. 2. Furan and pyran rings of D-galactose. The open chain formation of D-galactose is shown in the middle of the figure. The furanose (lower left) and pyranose (lower right) forms of D-galactose ( β is used in this example) are formed from the open chain structure. The generic furan (upper left) and pyran (upper right) ring for - -D-galactopyranose mations are also shown. β Fig. 4. Scheme showing the formation of the pyran ring. Step 1— Carbon 5 (square) of the open chain form of D-galactose rotates so that its hydroxyl group apposes carbon 1 (circle). Step 2—The double-bonded oxygen on carbon 1 is reduced and the hydroxyl group of carbon 5 is oxidized, leaving carbon 1 and the oxygen on carbon 5 each with one less than their preferred number of bonds. Step 3—A bond forms between carbon 1 and the oxygen of carbon 5 to yield β-D-galactopyranose. hexose sugars and carbon 2 in sialic acid. This carbon is termed the anomeric carbon, and the configurational difference occurs in the orientation of its hydroxyl group in relation to the oxygen on the anomeric- reference atom, which is carbon 5 in most hexoses. Generic hexose Nonose sugar, i.e., sialic acid Fig. 3. Carbon numbering in pyranose rings. Most of the common blood group sugars are 6-carbon (hexoses), except for sialic acid, which has 9 carbons (nonose).
Details
-
File Typepdf
-
Upload Time-
-
Content LanguagesEnglish
-
Upload UserAnonymous/Not logged-in
-
File Pages10 Page
-
File Size-