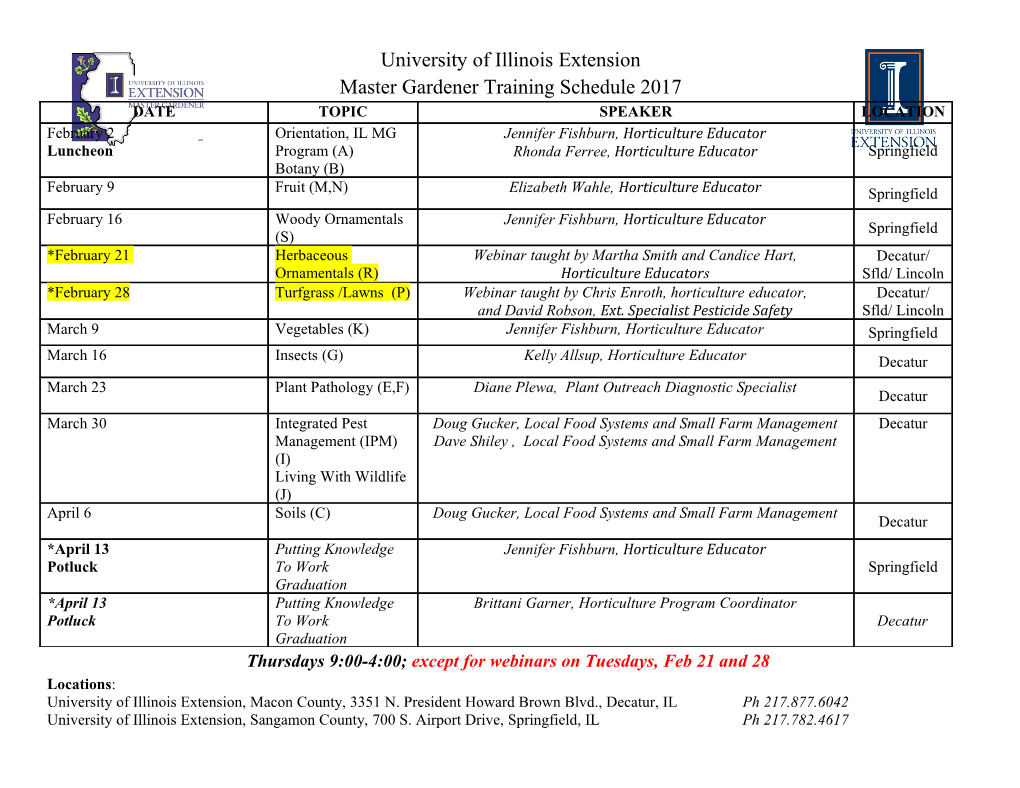
Hum Genet (2016) 135:1269–1278 DOI 10.1007/s00439-016-1720-4 ORIGINAL INVESTIGATION A 1.35 Mb DNA fragment is inserted into the DHMN1 locus on chromosome 7q34–q36.2 Alexander P. Drew1 · Anthony N. Cutrupi1,3 · Megan H. Brewer1,3 · Garth A. Nicholson1,2,3 · Marina L. Kennerson1,2,3 Received: 5 April 2016 / Accepted: 25 July 2016 / Published online: 3 August 2016 © Springer-Verlag Berlin Heidelberg 2016 Abstract Distal hereditary motor neuropathies predomi- for hereditary motor neuropathies and highlights the grow- nantly affect the motor neurons of the peripheral nervous ing importance of interrogating the non-coding genome for system leading to chronic disability. Using whole genome SV mutations in families which have been excluded for sequencing (WGS) we have identified a novel structural genome wide coding mutations. variation (SV) within the distal hereditary motor neuropa- thy locus on chromosome 7q34–q36.2 (DHMN1). The SV involves the insertion of a 1.35 Mb DNA fragment Introduction into the DHMN1 disease locus. The source of the inserted sequence is 2.3 Mb distal to the disease locus at chromo- The distal hereditary motor neuropathies (dHMN) are a some 7q36.3. The insertion involves the duplication of five group of progressive neurodegenerative disorders that pri- genes (LOC389602, RNF32, LMBR1, NOM1, MNX1) and marily affect the motor neurons of distal limbs without partial duplication of UBE3C. The genomic structure of affecting sensory neurons. The disorder is a length depend- genes within the DHMN1 locus are not disrupted by the ant neuropathy in which the longest nerves are initially insertion and no disease causing point mutations within affected. Chronic denervation leads to paresis and atro- the locus were identified. This suggests the novel SV is the phy of distal muscles, generally affecting lower limbs to a most likely DNA mutation disrupting the DHMN1 locus. greater extent than upper limbs. Distal HMN is a geneti- Due to the size and position of the DNA insertion, the cally heterogeneous group of diseases broadly classified gene(s) directly affected by the genomic re-arrangement by mode of inheritance, age of onset or additional clinical remains elusive. Our finding represents a new genetic cause features. Subtypes are defined by the specific gene mutated or a mapped disease locus (Drew et al. 2011; Rossor et al. 2012). Currently, there are 11 HMN types represented Electronic supplementary material The online version of this by 11 causative genes and five disease loci (Rossor et al. article (doi:10.1007/s00439-016-1720-4) contains supplementary 2012). material, which is available to authorized users. Genetic linkage analysis in a large Australian family * Alexander P. Drew with dHMN (F-54) mapped a disease locus to a 12 Mb [email protected] interval on chromosome 7q34–q36.2 (DHMN1) (Gopi- * Marina L. Kennerson nath et al. 2007). The family is described as dHMN type [email protected] I (DHMN1: OMIM %182960) or dHMN with pyramidal signs (Rossor et al. 2012). The neuropathy in this family 1 Northcott Neuroscience Laboratory, ANZAC Research Institute, University of Sydney, Concord, Sydney 2139, is characterised by juvenile onset and autosomal dominant Australia inheritance, with the lower limbs primarily affected. 2 Molecular Medicine Laboratory, Concord Hospital, Concord, Previous work to identify the pathogenic mutation in Sydney 2139, Australia F-54 has examined coding sequences of positional can- 3 Sydney Medical School, University of Sydney, Sydney, didate genes and copy number variations (CNV) in the Australia DHMN1 locus. Initially, Sanger sequencing of exons and 1 3 1270 Hum Genet (2016) 135:1269–1278 flanking intronic sequences ruled out mutations in 33 Isaac aligner (Illumina). Variant calls and annotations by candidate genes (Drew 2012; Gopinath et al. 2007). Next Macrogen were made using Isaac variant caller (Illumina), generation sequencing of targeted capture of the DHMN1 CNVseg (Ivakhno et al. 2010) and Manta (Chen et al. locus with the 454 platform (<20 read depth) and the Illu- 2016). Variant reports from Macrogen for SNP/INDEL and × mina Solexa platform (>220 read depth) in one affected CNV were further annotated using ANNOVAR software × individual and whole exome sequencing (WES) of an addi- (Wang et al. 2010). We selected SNP/INDELS within the tional two affected and one unaffected individuals (40 DHMN1 locus using the vcfintersect program from vcflib × depth) did not identify any disease candidate SNPs or (https://github.com/vcflib/vcflib). For in-depth investiga- INDELS (Drew 2012). Cytogenetic analysis using Trypsin tion of CNV we performed copy number variation analy- (GTL) banding did not detect any microscopic structural sis based on read depth of WGS data using the software variation (SV). Pathogenic copy number variation (CNV) CNVNator (Abyzov et al. 2011) with analysis of chromo- within the DHMN1 locus was excluded with an Agilent some 7 using a bin size of 250 bp. CNV were visualised SurePrint G3 custom CGH microarray specifically target- using CNVNator and ROOT (http://root.cern.ch). ing the DHMN1 locus (Drew 2012). This combination of sequencing and CNV analysis ruled out SNP, INDEL CNV analysis from WES and CNV mutations within the coding sequences of the DHMN1 locus and large microscopic SV. Retrospective CNV analysis of historic WES data from Despite this extensive investigation of genes, the F-54 was carried out using the software ExomeDepth DHMN1 locus remained genetically unsolved. Here we (Plagnol et al. 2012). In brief, WES was carried out by present analysis using whole genome sequencing (WGS) Axeq Technologies (Korea) using the TruSeq Exome with a focus on querying the patient DNA for SVs to iden- Library Prep Kit (Illumina) and sequenced using the tify the likely pathogenic mutation in family F-54 within HiSeq 2000 Sequencer (Illumina) as 100 bp paired-end the DHMN1 locus. reads to 40 read depth. Sequencing reads were assem- × bled to the Human Feb. 2009 (GRCh37/hg19) assembly using BWA-MEM (Li 2013) and bam files were sorted and Materials and methods indexed using Samtools (Li et al. 2009a). Each F-54 patient exome was compared to panel of six control exomes Study subjects matched from the same sequencing batch as per the Exo- meDepth guidelines. Patient ascertainment and blood sample collection was performed with informed consent according to protocols PCR and Sanger sequencing approved by the Sydney Local Health District Human Eth- ics Review Committee, Concord Repatriation General Hos- The genotyping assay using multiplex PCR was carried pital, Sydney, Australia (HREC/11/CRGH/105). Genomic out in a 10 μL reaction volume containing 1 MyTaq × DNA was extracted from whole blood using the PureGene HS Red Mix, 10 ng DNA template, 4 pmol each of for- kit (Qiagen). Clinical history, neurological examination and ward and reverse primer (A, C, D and F) and 8 pmol of the neurophysiology studies for members of the kindred have dual forward/reverse primer (B and E). Primer sequences been previously described (Gopinath et al. 2007). Four (5′–3′) include, Assay 1: Primer A, TCATGAACGCTG members from F-54 were selected for this study. The three TCGAATTT; Primer B, TCCAAAACCAAACAAGG affected individuals had different haplotypes for the normal ACA; Primer C, GTCACATGGTGAAGGCAGTC. Assay 2: chromosome at the DHMN1 locus and were therefore con- Primer D, TGTCCTTTGAATTCATCCATGT; Primer E, sidered to be as distantly related as possible. An unaffected TCAGCCTCTCAGGTTCAGG; Primer F, AACGGGG married-in parent of one of the affected individuals under- TCTCCAACAAATA. PCR cycling was performed using going WGS was chosen by DNA availability. a Mastercycler pro S (Eppendorf) and touchdown proto- col consisting of: initial denaturation 95 °C for 5 min; 11 Whole genome sequencing cycles of 95 °C for 30 s, 72 °C (reducing by 1.5 °C per cycle) for 30 s, and 72 °C for 30 s; 30 cycles of 95 °C for WGS was outsourced to Macrogen (South Korea). Sam- 30 s, 60 °C for 30 s, and 72 °C for 30 s; final extension at ple library construction was performed using the Illumina 72 °C for 5 min. PCR amplicons were prepared with stand- TruSeq Nano DNA sample preparation protocol. Libraries ard PCR protocols. Samples amplifying both wild type were sequenced using the Illumina HiSeq Ten Sequencer (wt) and mutant PCR amplicons were gel extracted using × to a target depth of 30 . Sequence reads were mapped the Isolate II PCR and gel kit (Bioline).and outsourced for × to the Human Feb. 2009 (GRCh37/hg19) assembly using sequence validation using the BigDye Terminator Cycle 1 3 Hum Genet (2016) 135:1269–1278 1271 Table 1 Rare nonsynonymous SNVs identified in the DHMN1 locus from WGS data after patient/control segregation analysis Gene Transcript ID Nucleotide Amino acid Frequency 1000 Frequency dbSNP142 variation variation genomes ExAC EPHB6 NM_004445 c.G364A p.G122S 0.003 0.007 rs8177173 GIMAP1–GIMAP5 NM_001199577 c.C499T p.R167C 0.006 0.006 rs9657892 TMEM176B NM_014020 c.C538T p.R180W 0.020 0.033 rs17256042 ABP1 NM_001272072 c.C995T p.S332F 0.053 0.060 rs1049742 ASIC3 NM_004769 c.C865T p.P289S 0.004 0.009 rs114024820 Sequencing protocols at the ACRF Facility, Garvan Insti- INDELs were selected. After filtering for rare variants with tute of Medical Research (Australia). 1000 Genomes frequency of 0.05 or less, 199 SNVs and 146 INDELs remained. Of these, five variants had a puta- Agarose gel electrophoresis tive pathogenic function (Table 1). However, these SNVs were all reported in the dbSNP142 and ExAC databases Agarose gels [1.5 % (w/v)] were prepared in 1 TAE in multiple populations, making them too common and × buffer with 6–10 % (v/v) SYBR Safe DNA gel stain. unlikely to be the pathogenic mutation in F-54. PCR products (3–5 μL) were size fractionated at 90 V 1 for 40 min (40 V cm− ).
Details
-
File Typepdf
-
Upload Time-
-
Content LanguagesEnglish
-
Upload UserAnonymous/Not logged-in
-
File Pages10 Page
-
File Size-