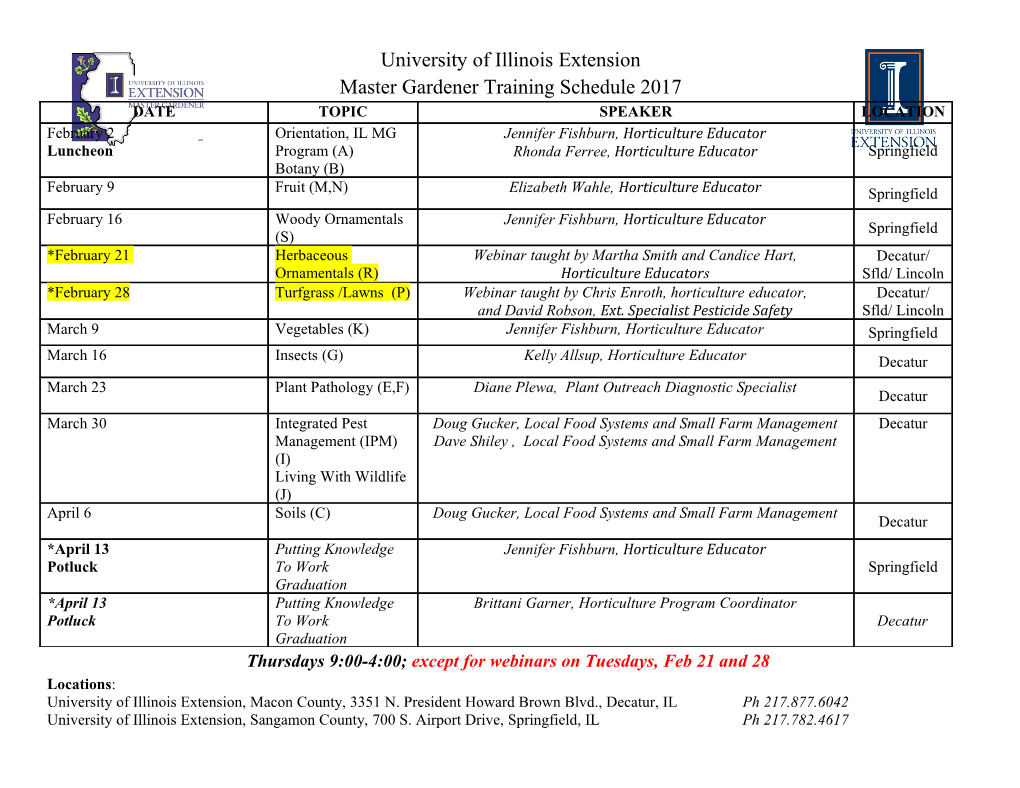
Gamma Radioactivity Gamma radioactivity is composed of electromagnetic rays. It is distinguished from x-rays only by the fact that it comes from the nucleus. Most gamma rays are somewhat higher in Index energy than x-rays and therefore are very penetrating. It is the most useful type of radiation for medical purposes, but at the same time it is the most dangerous because of its ability to penetrate large thicknesses of material. Go Back HyperPhysics***** Nuclear R Nave After a decay reaction, the nucleus is often in an excited state. This means that the decay has resulted in producing a nucleus which still has excess energy to get rid of. Rather than emitting another beta or alpha particle, this energy is lost by emitting a pulse of electromagnetic radiation called a gamma ray. The gamma ray is identical in nature to light or microwaves, but of very high energy. Like all forms of electromagnetic radiation, the gamma ray has no mass and no charge. Gamma rays interact with material by colliding with the electrons in the shells of atoms. They lose their energy slowly in material, being able to travel significant distances before stopping. Depending on their initial energy, gamma rays can travel from 1 to hundreds of meters in air and can easily go right through people. It is important to note that most alpha and beta emitters also emit gamma rays as part of their decay process. However, there is no such thing as a “pure” gamma emitter. Important gamma emitters including Technetium-99m which is used in nuclear medicine, and Cesium-137 which is used for calibration of nuclear instruments. Other Radioactive Processes While the most common types of radioactive decay are by alpha, beta, and gamma radiation, several other varieties of radioactivity occur: Electron capture: A parent nucleus may capture one of its own electrons and emit a neutrino. This is exhibited in the potassium-argon decay. Index Positron or positive beta decay: Positron emission is called beta decay because the characteristics of electron or positron decay are similar. They both show a characteristic energy spectrum because of the emission of a neutrino or antineutrino. Internal conversion is the use of electromagnetic energy from the nucleus to expel an orbital electron from the atom. Go Back HyperPhysics***** Nuclear R Nave Electron Capture Electron capture is one form of radioactivity. A parent nucleus may capture one of its orbital electrons and emit a neutrino. This is a process which competes with positron emission and has the same effect on the atomic number. Most commonly, it is a K-shell electron which is captured, and this is referred to as K-capture. A typical example is Index In the middle range of the periodic table, those isotopes which are lighter than the most stable isotopes tend to decay by electron capture, and those heavier decay by negative beta decay. An example of this pattern is seen with silver isotopes, with two stable isotopes plus one of lower mass which decays by electron capture and one of heavier mass which decays by beta emission. Cobalt-57 Go Back HyperPhysics***** Nuclear R Nave Internal Conversion Internal conversion is another electromagnetic process which can occur in the nucleus and which competes with gamma emission. Sometimes the multipole electric fields of the nucleus interact with orbital electrons with enough energy to eject them from the atom. This process is not the same as Index emitting a gamma ray which knocks an electron out of the atom. It is also not the same as beta decay, since the emitted electron was previously one of the Reference orbital electrons, whereas the electron in beta decay is produced by the decay Krane of a neutron. Sec 10.6 An example used by Krane is that of 203Hg, which decays to 203Tl by beta emission, leaving the 203Tl in an electromagnetically excited state. It can proceed to the ground state by emitting a 279.190 keV gamma ray, or by internal conversion. In this case the internal conversion is more probable. Since the internal conversion process can interact with any of the orbital electrons, the result is a spectrum of internal conversion electrons which will be seen as superimposed upon the electron energy spectrum of the beta emission. The energy yield of this electromagnetic transition can be taken as 279.190 keV, so the ejected electrons will have that energy minus their binding energy in the 203Tl daughter atom. Electron emissions from the Hg-203 to Tl-203 decay, measured by A. H. Wapstra, et al., Physica 20, 169 (1954). At higher resolution, the internal conversion electrons from the L, M and N shells can be resolved. Z. Sujkowski, Ark. Fys. 20, 243 (1961). At even higher resolution, the three L shells can be resolved. From C. J. Herrlander and R. L. Graham, Nucl. Phys. 58, 544 (1964). The resolution of the electron detection is good enough that such internal conversion electron spectra can be used to study the binding energies of the electrons in heavy atoms. In this case, the measured electron energies can be subtracted from the transition energy as indicated by the gamma emission, 279.190 keV. Binding energies for 203Tl K 85.529 keV LI 15.347 keV LII 14.698 keV LIII 12.657 keV M 3.704 keV In addition to information from the internal conversion electrons about the binding energies of the electrons in the daughter atom, the relative intensities of these internal conversion electron peaks can give information about the electric multipole character of the nucleus. Go Back HyperPhysics***** Nuclear R Nave .
Details
-
File Typepdf
-
Upload Time-
-
Content LanguagesEnglish
-
Upload UserAnonymous/Not logged-in
-
File Pages7 Page
-
File Size-