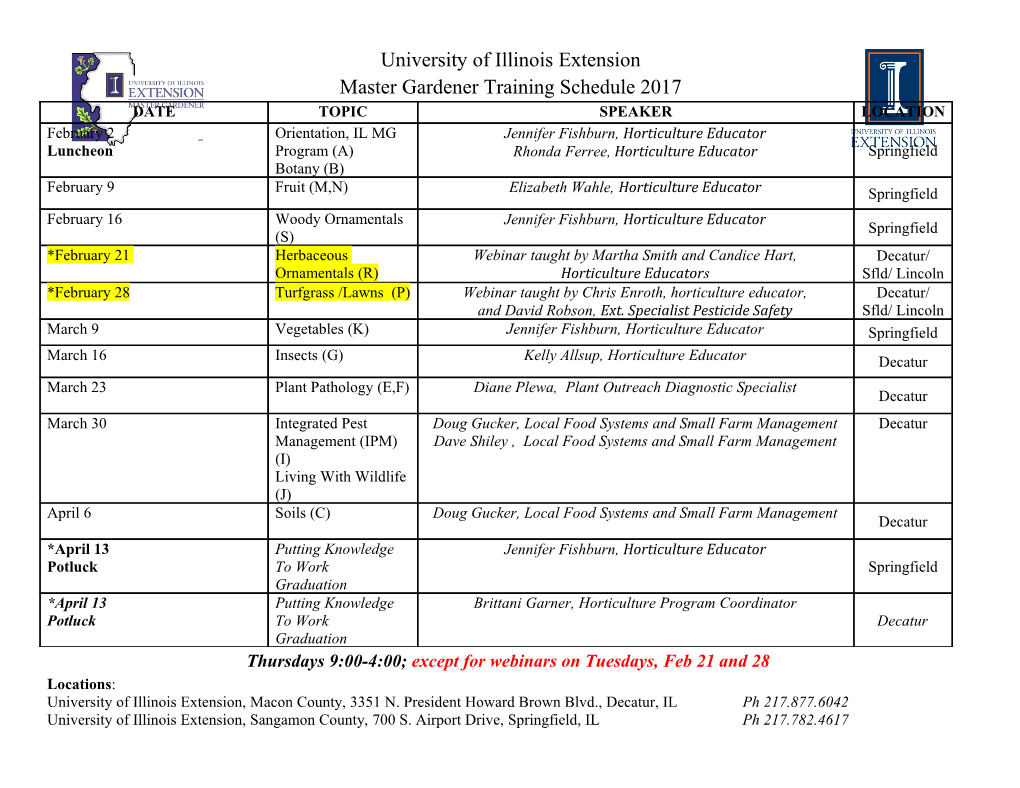
PUBLICATIONS Paleoceanography RESEARCH ARTICLE Regional and global benthic δ18O stacks for the last 10.1002/2016PA003002 glacial cycle Key Points: Lorraine E. Lisiecki1 and Joseph V. Stern1 • Volume-weighted global benthic δ18O stack for 0-150 ka on speleothem age 1Department of Earth Science, University of California, Santa Barbara, California, USA model • Benthic δ18O responds to insolation with a mean time constant of 3-8 kyr • Regional stacks allow for diachronous Abstract Although detailed age models exist for some marine sediment records of the last glacial cycle 18 18 benthic δ O change (0–150 ka), age models for many cores rely on the stratigraphic correlation of benthic δ O, which measures ice volume and deep ocean temperature change. The large amount of data available for the last 18 Supporting Information: glacial cycle offers the opportunity to improve upon previous benthic δ O compilations, such as the “LR04” • Supporting Information S1 global stack. Not only are the age constraints for the LR04 stack now outdated but a single global alignment • Table S1 target neglects regional differences of several thousand years in the timing of benthic δ18O change during • Data Set S1 18 • Data Set S2 glacial terminations. Here we present regional stacks that characterize mean benthic δ O change for 8 ocean • Data Set S3 regions and a volume-weighted global stack of data from 263 cores. Age models for these stacks are based on radiocarbon data from 0 to 40 ka, correlation to a layer-counted Greenland ice core from 40 to 56 ka, and Correspondence to: correlation to radiometrically dated speleothems from 56 to 150 ka. The regional δ18O stacks offer better L. E. Lisiecki, [email protected] stratigraphic alignment targets than the LR04 global stack and, furthermore, suggest that the LR04 stack is biased 1–2 kyr too young throughout the Pleistocene. Finally, we compare global and regional benthic δ18O responses with sea level estimates for the last glacial cycle. Citation: Lisiecki, L. E., and J. V. Stern (2016), Regional and global benthic δ18O stacks for the last glacial cycle, 1. Introduction Paleoceanography, 31, doi:10.1002/ – 2016PA003002. The large number of paleoclimate records that exist for the last glacial cycle (0 150 ka) have the potential to greatly improve our understanding of Earth’s climate responses on both millennial and orbital time scales. Received 8 JUL 2016 Most notably, this time range covers two glacial terminations and many millennial-scale climate changes. Accepted 21 SEP 2016 Analysis of paleoclimate records during this time period can yield a wealth of information about climate Accepted article online 23 SEP 2016 response times, feedback, stability, and sensitivity to external (orbital) forcing and atmospheric greenhouse gas levels. However, understanding these responses in detail requires high-resolution records of many differ- ent parts of the climate system with very precise age control. Some climate archives are well suited to dating, for example by annual layering or radiometric decay. However, ocean sediment cores are difficult to date precisely despite offering the potential to reconstruct cli- mate responses over more than half of Earth’s surface. Radiocarbon offers relatively good age control for the last 40 kyr but is costly and can still have calendar age uncertainties of more than 1000 years due to calibra- tion and reservoir age uncertainty [e.g., Waelbroeck et al., 2001; Stern and Lisiecki, 2013; Reimer et al., 2013]. Beyond 40 ka, sediment cores are generally dated by correlating a paleoclimate proxy to either a better dated climate archive (e.g., ice cores or speleothems) or to changes in Earth’s orbital configuration (i.e., “orbital tun- ing”). The accuracy of stratigraphically correlated age models depends on both the accuracy of the target age model and the extent to which the proxy measured in the core is physically linked to the selected target [Govin et al., 2015]. The vast majority of marine sediment cores have age models based on stratigraphic alignment of the oxygen isotope composition (δ18O) of benthic foraminiferal calcite, which measures changes in ice volume and deep ocean temperature. Traditionally, benthic δ18O has been assumed to change synchronously throughout the ocean despite a 1–2 kyr mixing time for the deep ocean [e.g., Pisias et al., 1984; Imbrie et al., 1992]. Therefore, a core’s benthic δ18O is often aligned to another core with better age control (e.g., 14C, dated ash layers, or mag- netic anomalies) or to a global δ18O stack (average) [e.g., Imbrie et al., 1984; Lisiecki and Raymo, 2005]. If δ18O changes were synchronous, alignment of all records to a single δ18O target, such as a stack, would allow mutually consistent comparison of the timing of all climate responses recorded in marine sediment cores. However, radiocarbon age models reveal differences of up to 4 kyr in the timing of benthic δ18O change dur- ©2016. American Geophysical Union. ing the last glacial termination (T1) [Skinner and Shackleton, 2005; Stern and Lisiecki, 2014] and likely other late 18 All Rights Reserved. Pleistocene terminations [Lisiecki and Raymo, 2009]. Although alignment to a global δ O target appears ill LISIECKI AND STERN LAST GLACIAL CYCLE BENTHIC δ18O1 Paleoceanography 10.1002/2016PA003002 advised during glacial terminations, the timing of δ18O change may be synchronous to within a few hundred years in certain regions of the ocean bathed by a relatively uniform water mass [Waelbroeck et al., 2011; Stern and Lisiecki, 2014]. Thus, we develop eight regional benthic δ18O stacks for the last glacial cycle, which we suggest make better alignment targets than a single global stack. We estimate age differences between regions and account for the considerable uncertainty of these estimated lags. Finally, we construct a volume-weighted global stack for comparison with ice volume (sea level) records and the commonly used “LR04” global benthic stack [Lisiecki and Raymo, 2005]. A second goal of this work is to improve the absolute age estimates for δ18O stratigraphy, accompanied by explicit age uncertainty estimates. The age constraints used in the LR04 stack for the last glacial cycle are now largely outdated. Only one radiocarbon-dated core [Waelbroeck et al., 2001] constrains T1, an outdated Greenland ice core age model [Johnsen et al., 1992] is used for 20–120 ka, and uncertain coral-based sea level estimates [Bard et al., 1990] constrain the penultimate termination (T2). For the new stacks, we use regional radiocarbon compilations from 0 to 40 ka [Stern and Lisiecki, 2014], develop millennial-scale correlations between North Atlantic cores, a layer-counted Greenland ice core record from 40 to 56 ka, and radiometrically dated speleothems from 56 to 150 ka. These improved benthic age δ18O estimates have implications for the entire Pleistocene because the orbital tuning time constant used in the LR04 age model was derived from evaluation of benthic δ18O responses from 0 to 150 ka. 2. Age Models for the Last Glacial Cycle 2.1. Speleothems The most precise age models that span the last glacial cycle come from U-series-dated speleothem calcite δ18O records. Chinese speleothem records are often interpreted as proxies for summer Asian monsoon strength, with more negative values indicating stronger monsoon [e.g., Wang et al., 2001]. We created a com- posite Chinese speleothem record by combining the Hulu and Sanbao records (Figure 1a) with 1.6‰ added to the Sanbao values to correct for fractionation effects due to elevation difference [Wang et al., 2008]. The age model for this combined Hulu/Sanbao δ18O record is constrained by approximately 100 radiometric dates, with age uncertainty less than ±2000 years for the entire interval and around ±100 years (2σ) during T2 due to focused efforts. The pronounced weak monsoon interval (WMI) associated with T2 occurs between 136 and 129 ka [Cheng et al., 2009]. Several precisely dated stalagmites are available from caves located at the northern rim of the Alps (NALPS), a region whose climate is tightly coupled with Greenland and the North Atlantic [Boch et al., 2011]. Given the more direct climate linkage between the Alps and the North Atlantic and the abrupt changes recorded in the NALPS speleothems, we use these records where available (75–110 ka). 2.2. Greenland Ice Cores The current reference record for Greenland is North Greenland Ice Core Project (NGRIP) δ18O[North Greenland Ice Core Project Members, 2004] on the layer-counted Greenland Ice Core Chronology 2005 (GICC05) chronol- ogy back to 60 ka [Svensson et al., 2008] merged with the NGRIP2004 [North Greenland Ice Core Project Members, 2004] age model shifted by 750 years from 60 to 122 ka [Wolff et al., 2010]. The NGRIP2004 age model is based on correlating to another Greenland ice core back to 105 ka (GRIP on the ss09sea chronology [Johnsen et al., 2001]) and to the Antarctic Vostok ice core from 105 to 122 ka [Petit et al., 1999]; both of which are derived from ice flow and snow accumulation models. With NGRIP δ18O on the combined age model (referred to as “GICC05modelext”), light isotopic intervals (stadials) in Greenland consistently occur during heavy isotopic intervals (weak monsoons) in China (Figure 1). Thus, the GICC05modelext and Hulu/Sanbao speleothem age models, which are completely independent, appear to be in excellent agreement over the entire 0–122 ka interval. The North Greenland Eemian Ice Drilling (NEEM) ice core provides an undisturbed section from about 115 to 129 ka, but better absolute age constraints for this core are still needed [NEEM Community Members, 2013]. 2.3. Antarctic Ice Cores Recent Antarctic ice core age models are all fixed to GICC05 for 0–60 ka and based on combinations of gla- ciological modeling and “a wide range of relative and absolute gas and ice stratigraphic markers” [Veres et al., 2013] for older portions.
Details
-
File Typepdf
-
Upload Time-
-
Content LanguagesEnglish
-
Upload UserAnonymous/Not logged-in
-
File Pages27 Page
-
File Size-