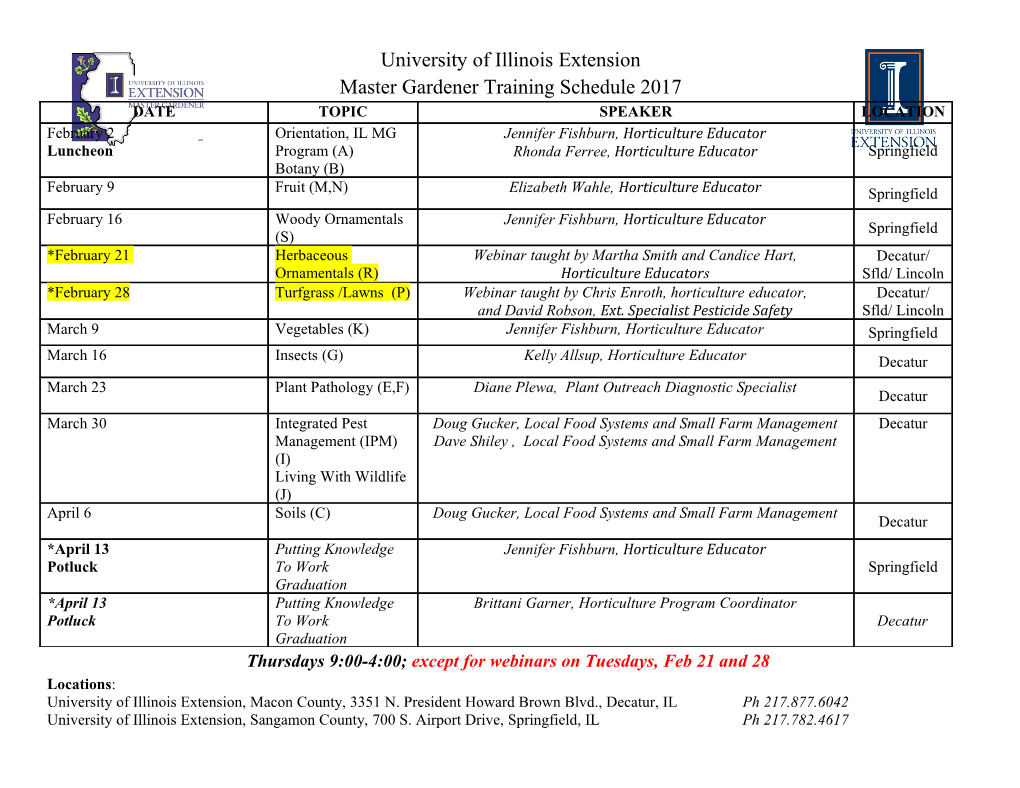
Deep Space Industries Inc. In-Space Production NNX15AL85G of Storable Propellants NIAC Phase I Final Report for NNX15AL85G: In-Space Production of Storable Propellants Deep Space Industries Inc. John S. Lewis, P. I. 1 March 2016 Deep Space Industries Inc. In-Space Production NNX15AL85G of Storable Propellants Table of Contents Overview and Conclusions ............................................................................................................................ 1 Introduction .................................................................................................................................................. 5 1. Available Feedstock on C-cadre Asteroids ................................................................................................ 5 2. Energy Requirements for Heating Feedstock ........................................................................................... 6 3. Recovered Water and its Uses ................................................................................................................ 16 4. Electrolysis of Water for STP or Direct Combustion .............................................................................. 17 5. Electrolysis of Water for Manufacture of Storables .............................................................................. 19 6. Where Should we Make Propellants? ..................................................................................................... 20 7. Storage and Transportation of Water and Carbon Dioxide ................................................................... 20 8. Methanol/Dimethyl Ether Synthesis ...................................................................................................... 22 9. Conventional (Earthside) H2O2 Synthesis ................................................................................................ 24 10. Space-based direct synthesis of H2O2 ................................................................................................... 25 11. Long-Term Storage of Hydrogen Peroxide ............................................................................................ 26 12. Effect of Peroxide Degradation on Propellant Performance ................................................................ 28 13. Freezing and Distillation Behavior of Hydrogen Peroxide ................................................................... 28 14. Raw Materials Requirements for Storable Propellant Synthesis .......................................................... 30 15. DME/H2O2 Combustion ........................................................................................................................ 32 16. Schematic Processing System Architecture on an Asteroid................................................................. 33 17. Schematic Processing System Architecture in Earth Orbit .................................................................. 37 18. Hydrogen peroxide as a monopropellant ............................................................................................ 42 19. Applications of this Propellant Manufacturing Scheme: the Mars System and the Moon ................. 42 20. Mission Opportunities for Use of Storable Space-based Propellants .................................................. 43 20.1 Transportation from NEAs to Earth’s vicinity. ................................................................................ 44 20.2 Capture and delivery to HEEO Processing Facility orbit via single lunar swingby. ......................... 47 20.3 Selection of the HEEO Processing and Storage Facility Orbit ......................................................... 50 20.4 Delivery of processed propellants to customer orbits. .................................................................. 51 21. Synergy with New-Generation Low-Cost Earth-Launch Technologies ................................................ 55 22. Terrestrial applications of improved H2O2 syntheses ........................................................................... 55 References .................................................................................................................................................. 56 1 Deep Space Industries Inc. In-Space Production NNX15AL85G of Storable Propellants List of Tables Table 1. Potential fuel/oxidizer pairs compared to feedstock composition ............................................... 31 Table 2. Heats of combustion for assorted fuel/oxidizer pairs .................................................................. 32 Table 3. Analysis of producing different fuels for use with HTP. ............................................................... 41 Table 4. ΔV costs (impulsively modeled) for delivery of HEEO materials to markets in other orbits. ....... 51 List of Figures Fig. 1 Decomposition pressures of various hydrous mineral phases. ....................................................... 10 Fig. 2 Projection of the Fe-S-O phase diagram on the p(s2)-p(O2) plane at 800 K. .................................. 12 Fig. 3 Projection of the Fe-S-O phase diagram on the p(s2)-p(O2) plane at 1000 K. ................................. 13 Fig. 4 The equilibrium stability field of CO2 clathrate hydrate ................................................................. 21 Fig. 5 Rate of decomposition of HTP H2O2 in Al-alloy tanks. ................................................................... 27 Fig. 6 Equilibrium phase diagram of the H2O2-H2O system on the p(H2O2)-p(H2O) plane. ................... 29 Fig. 7 Analysis of at-the-asteroid processing identified 13 major phases ................................................. 35 Fig. 8 The synthesis of HTP and a fuel (TBD) will be conducted in Earth orbit. ......................................... 40 Fig. 9 Ballistic Trajectory between 2009 HC and Earth. ............................................................................. 46 Fig. 10 STP transfer between 2009 HC and Earth. Isp = 200 s, Thrust = 45N @ 1 AU ................................. 46 Fig. 11 STP transfer between 1998 KY26 and Earth. Isp = 200 s, Thrust = 80N @ 1 AU .............................. 47 Fig. 12. Lunar swingby trajectory. .............................................................................................................. 48 Fig. 13 “Porkchop” Plot Showing Mission Opportunity in terms of ΔV ...................................................... 54 2 Deep Space Industries Inc. In-Space Production NNX15AL85G of Storable Propellants Overview and Conclusions Our exploration of the feasibility of in-space production of storable propellants from resources available on asteroids, and also on Mars and the Moon, has considered the process of sample acquisition; the energy requirements for heating and volatile release; the thermodynamic behavior of gas release from minerals and organic polymers containing hydrogen, oxygen, carbon, sulfur, and nitrogen; purification of the released water and carbon dioxide; the storage and transportation of these materials in the condensed state; synthesis of fuels including methanol and dimethyl ether (DME); and the co-production, concentration, stability, and storage of the complementary oxidizer high-test hydrogen peroxide (HTP). We call attention to the ability of the storable propellant/oxidizer combination of DME and HTP to carry out deep-space missions and to perform retrieval and relocation of any and all space-derived resources, such as retrieving asteroidal metal to high Earth orbit. This study’s analyses are based on returning resources to a storage/processing/dispensing facility in a Highly Eccentric Earth Orbit (HEEO) with a perigee above geosynchronous orbit and an apogee approach or beyond the Moon’s orbit. The methane/LOX option, notable for good engine performance, has not been included in this study because our scope includes only fully storable propellants. This work in Phase I has led to a number of conclusions. These are: 1. Thermodynamic theory shows that extraction of water and carbon dioxide from carbonaceous (C-type) near-Earth asteroids by means of direct solar heating is feasible and efficient. This is in agreement with experiments carried out by Joel Sercel in an independent NIAC Phase I project, using both CM type meteorite material and a C-type asteroid simulant that we have developed at DSI and the University of Central Florida under an SBIR grant running concurrently with this (and his) NIAC grant. 2. The same theory also predicts that attempts at full extraction of volatiles from a C asteroid will require calciner temperatures of at least 700 K, at which temperature not only does the native organic polymer in the C asteroid material react with magnetite (Fe3O4) to generate carbon dioxide and water vapor, but also these gases react with coexisting sulfide and sulfate minerals to release copious amounts of sulfur dioxide. This prediction has also been qualitatively verified by Joel Sercel’s work on meteorite and DSI simulant materials. At these temperatures, release of H, C, O, N, and S produces gases amounting to about 40% of the total mass of the asteroidal material. 3. The principal sulfur gas released, sulfur dioxide, is a source of some concern for several reasons. It is a toxic and offensively odorous gas that must be removed from water intended for life-support or hydroponic use. It also spontaneously generates elemental sulfur and sulfuric acid, highly undesirable materials that can corrode or clog water-handling systems
Details
-
File Typepdf
-
Upload Time-
-
Content LanguagesEnglish
-
Upload UserAnonymous/Not logged-in
-
File Pages61 Page
-
File Size-