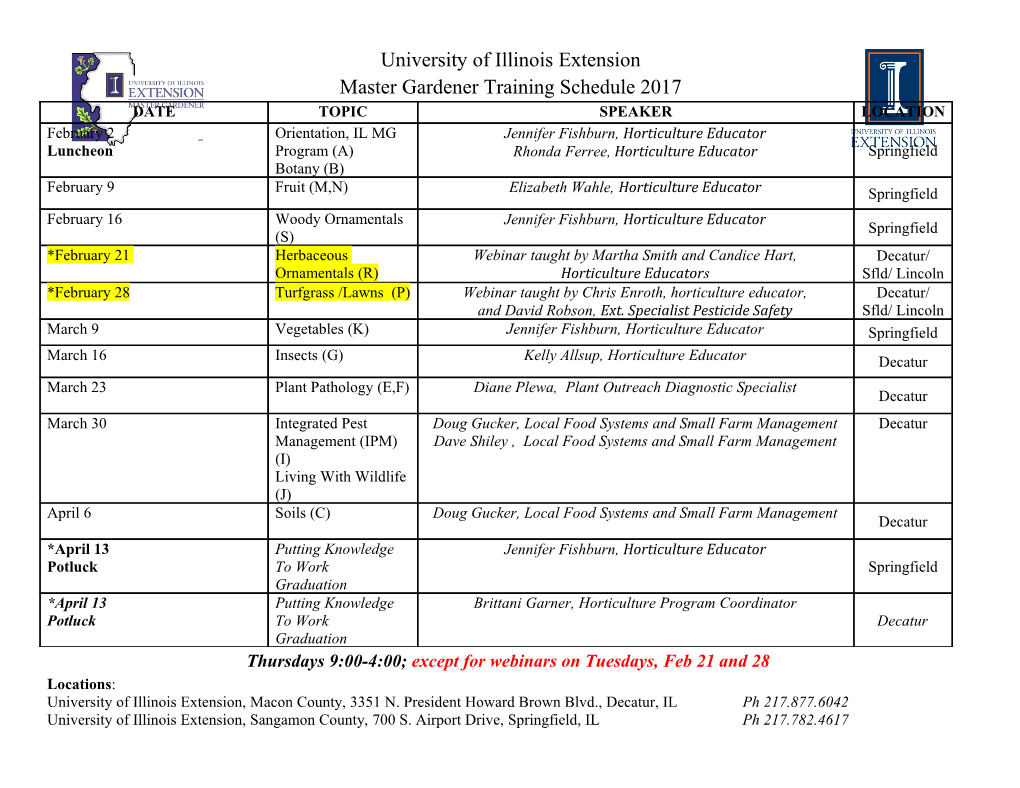
Understanding Microstructural Evolution in Mixed Metal-Oxide in Mixed Evolution MicrMicrostructural Understanding Silsesquioxane Glasses Through Wet-Chemical Synthesis Wet-Chemical Glasses Through SilsesquioSilsesquioxane Understanding Microstructural Uitnodiging xane Glasses Thr xane Evolution in Mixed Metal-Oxide ostructural Evolution in Mix Evolution ostructural Silsesquioxane Glasses Through Graag nodig in u en uw partner uit voor het bijwonen van de ough Wet-Chemic Wet-Chemical Synthesis openbare verdediging van mijn proefschrift: Understanding Microstructural Evolution in Mixed Metal-oxide al Synthesis Silsesquioxane Glasses Through ed Metal-Oxide Wet-Chemical Synthesis op vrijdag 28 maart 2014 om 12:45 h in de prof. dr. G Berkhoff-zaal (Collegezaal 4) van het gebouw de Waaier van de Universiteit Twente. Voorafgaand zal ik om 12:30 h mijn proefschrift in het kort toelichten. Aanluitend aan de verdediging is er een receptie ter plaatse Rogier Besselink2014 Rogier Vanaf 20:30 h bent u eveneens van harte welkom op het feest op Boerderij Bosch Rogier Besselink [email protected] Paranimfen: Tomasz Stawski Wouter Maijenburg ISBN: 978-90-365-3637-0 Rogier Besselink UNDERSTANDING MICROSTRUCTURAL EVOLUTION OF MIXED METAL OXIDE SILSESQUIOXANE GLASSES THROUGH WET-CHEMICAL SYNTHESIS Rogier Besselink PhD committee Chairman and secretary: Prof. dr. ir. J.W.M. Hilgenkamp University of Twente Supervisor: Prof. dr. ir. J.E. ten Elshof University of Twente Members: Prof. dr. L. van Wüllen University of Ausburg Prof. dr. A. Nijmeijer University of Twente Prof. dr. ing. D.H.A Blank University of Twente Prof. dr. A.J.A. Winnubst University of Science and Technology of China, Hefei / University of Twente Referent: Dr. A. Petukhov Utrecht University Cover: Simulated 2D-small angle X-ray pattern of a mixture of correlated spherical agglomerates with branched polymeric agglomerates. The molecule on the backside represents: Nb 8O10 (OEt) 20 . The work in this thesis was carried out at the Inorganic Materials Science Group at the faculty of Science and Technology and the MESA+ Institute for Nanotechnology, University of Twente, P.O. Box 217, 7500 AE, Enschede. This research was financially supported by (STW) the National Institute of Technical Sciences. R. Besselink: Understanding microstructural evolution of mixed metal oxide silses- quioxane glasses through wet-chemical synthesis. PhD Thesis, University of Twente, Enschede, The Netherlands ISBN: 978-90-365-3637-0 DOI: 10.3990/1.9789036536370 Printed by: Gildeprint drukkerijen, Enschede, The Netherlands Copyright ©2014 by: R. Besselink UNDERSTANDING MICROSTRUCTURAL EVOLUTION OF MIXED METAL OXIDE SILSESQUIOXANE GLASSES THROUGH WET-CHEMICAL SYNTHESIS PROEFSCHRIFT ter verkrijging van de graad van doctor aan de Universiteit Twente, op gezag van de rector magnificus, Prof. dr. H. Brinksma, volgens besluit van het College voor Promoties in het openbaar te verdedigen op vrijdag 28 maart 2014 om 12:45 uur door Rogier Besselink geboren op 28 februari 1983 te Doetinchem Dit proefschrift is goedgekeurd door de promotor Prof. dr. J.E. ten Elshof Table of Contents Chapter 1. Introduction 9 1.1) Sol-Gel Chemistry 10 1.2) Sol-Gel Processing 22 1.3) Porous Inorganic Membranes 26 1.4) The Scope of the Thesis and Outline 30 1.5) References 32 Chapter 2. Theory of Small Angle Scattering 39 2.1) Form Function 41 2.2) Structure function 43 2.3) Polydisperse Systems 51 2.4) Non-particulate systems 52 2.5) References 53 Chapter 3. Rapid early stage Schultz-Zimm distributed 57 mass-fractalic growth studies on niobia/silsesquioxane mixtures 3.1) Abstract 58 3.2) Introduction 58 3.3) Experimental section 60 3.4) Theoretical Background 63 3.5) Results and Discussion 72 3.6) Conclusions 78 3.7) References 78 Chapter 4. Evolution of microstructure in mixed niobia- 83 hybrid silica thin films from sol-gel precursors 4.1) Abstract 84 4.2) Introduction 84 4.3) Experimental Section 86 4.4) Derivation of I(q) 88 4.5) Results and Discussion 96 4.6) Conclusions 113 4.7) References 114 S4.) Supporting Information 121 S4.1) SAXS- Porod regime 122 S4.2) Nitrogen Sorption Measurements 123 S4.3) References 124 Chapter 5. Extent of niobia/silsesquioxane intermixing 125 by solid state MAS-NMR 5.1) Abstract 126 5.2) Introduction 126 5.3) Experimental Section 128 5.4) Results and Discussion 130 5.5) Conclusions 138 5.6) References 139 Chapter 6. Crystallized Nb 8O10 (OEt) 20 from 143 condensation of niobium ethoxide and acetone that exhibits remarkable anisotropy in thermal expansion 6.1) Abstract 144 6.2) Introduction 144 6.3) Experimental Section 146 6.4) Results and Discussion 147 6.5) Conclusions 157 6.6) References 157 S6.) Supporting information 161 S6.1) Extended lists of bond lengths and angles 162 S6.2) References 165 Chapter 7. A novel malonamide bridged silsesquioxane 167 precursor for enhanced dispersion of transition and lanthanide metal ions in hybrid silica membranes 7.1) Abstract 168 7.2) Introduction 168 7.3) Experimental Section 171 7.4) Results and Discussion 177 7.5) Conclusions 199 7.6) References 200 Chapter 8. Conclusions and Outlook 207 8.1) General Conclusions 208 8.2) Additional observations and remarks 209 8.3) Future Perspective and outlook 210 8.4) References 212 Summary 213 Samenvatting 217 Acknowledgement 221 Dankwoord 225 1. Introduction Chapter 1 1.1. Sol-Gel chemistry 1.1.1. The chemistry of silicon alkoxides Amorphous silicon oxides can be prepared via polymerisation reactions of silicon alkoxides with water. The silicon alkoxides initiate via a hydrolysis reaction (Scheme 1.1) and subsequently propagate via a number of different reaction processes (Scheme 1.2) [1-3]. Propagation mainly occurs via condensation reactions that either involves the elimination of water (oxolation) or the elimination of alcohol (alkoxolation). (RO) 3Si OR + H2O (RO) 3Si OH ROH Scheme 1.1. Hydrolysis of silicon alkoxides H alkoxolation (RO) 3Si O + O Si(OR) 3 (RO) 3Si O Si(OR) 3 + ROH R H oxolation (RO) 3Si O + O Si(OR) 3 (RO) 3Si O Si(OR) 3 + H2O H Scheme 1.2. Condensation of siliconolation alkoxides In polar solvents, the polymerisation reactions pursue an equilibrium that strongly depends on the reaction conditions. Different structures and morphologies were obtained by tuning suitable reaction conditions, which makes these sol-gel techniques very attractive for a wide range of materials. Propagation is in general terminated by limited mobility of reactive species that are entrapped into network and by reduction of reactive monomer species. The final product often contains a certain content of alkoxide groups can be removed by calcination. Silicon alkoxides are hydrophobic compounds with a low polarity. This is partly caused by the higher electronegativity of silicon (1,74) in comparison to early transition metal ions in metal alkoxides. A low polarity of silicon alkoxides corresponds with a very low solubility of silicon alkoxides in water [3]. In addition negative partial charge on oxygen of the alkoxide group is further stabilized by a 10 Introduction mesomeric interaction between occupied 2p-orbitals of oxygen and empty 3d-orbitals of silicon as illustrated in Scheme 1.3 [4]. In contrast to other metal alkoxides, silicon alkoxides are very weak Lewis acids/bases, which corresponds with a hydrolysis rate in water that is orders of magnitudes smaller in comparison to most metal alkoxides [1, 2]. : + - R O Si(OR) 3 RO Si(OR) 3 Scheme 1.3. Overlap of between O 3p and empty Si 3d orbitals The pH has a strong effect on the hydrolysis of silicon, while it determines the stability and the type of transition state and consequently it strongly affect the activation energy of hydrolysis. At a pH below the isoelectric point (IEP = 2.2) hydrolysis occurs via an acid catalysed mechanism and above IEP via a base catalysed mechanism [1]. The polarity of the silicon oxide bond is partly determined by the type of alkoxide. This can be explained by an inductive effect. The longer the alkane chain of the alkoxide, the stronger is the electron propulsion of this chain which results in a stabilisation of the negative charge of oxygen. Therefore the rate of hydrolysis decreases in the sequence: Si(OC 2H5)4> Si(OC 4H9)4> Si(OC 9H19 )4 [2]. Steric hindrance can also play a role in the reactivity of alkoxide and the hindrance will increase with increase of the alkyl chain length. Thus, the observed reduction of hydrolysis rate as a function of alkyl chain length is also explained in terms of steric hindrance. The alkoxide ligands of silicon alkoxide can exchange by a reaction with different alcohols. However, due to the low polarity of the silicon alkoxide bond, dissociation rarely occurs at room temperature and the exchange process takes a number of days. For example: It takes 5 days before 26% of Si(OMe) 4 have exchanged a single methoxide ligand for an ethoxide ligand in ethanol (OMe:EtOH = 1:1) [5]. The dissociation of alkoxide ligands depends on the charge on the partial charge of the silicon atom. In the presence of either Bronsted or Lewis acids the oxigen atoms become more positively charged and therefore the strength of the silicon oxide bond decreases. For acid catalysed systems 90% of the silicon methoxide precursors are partly substituted within 2 hours [5]. A similar increase was 11 Chapter 1 observed for Lewis catalysed hydrolysis of silicon methoxide, with either titanium(IV) isopropoxide or vanadium (V) oxy tri-isopropoxide. 1.1.1.a. Hydrolysis of silicon alkoxides: The basic catalysed mechanism occurs via a nucleophilic substitution according to an S N2-mechanism, which involves only one intermediate state (Scheme 1.4). The reaction involves the formation of a penta-coordinated negatively charged intermediate. The stability of this intermediate is dependent on the inductive effect of the substituents that surround the silicon atom.
Details
-
File Typepdf
-
Upload Time-
-
Content LanguagesEnglish
-
Upload UserAnonymous/Not logged-in
-
File Pages230 Page
-
File Size-