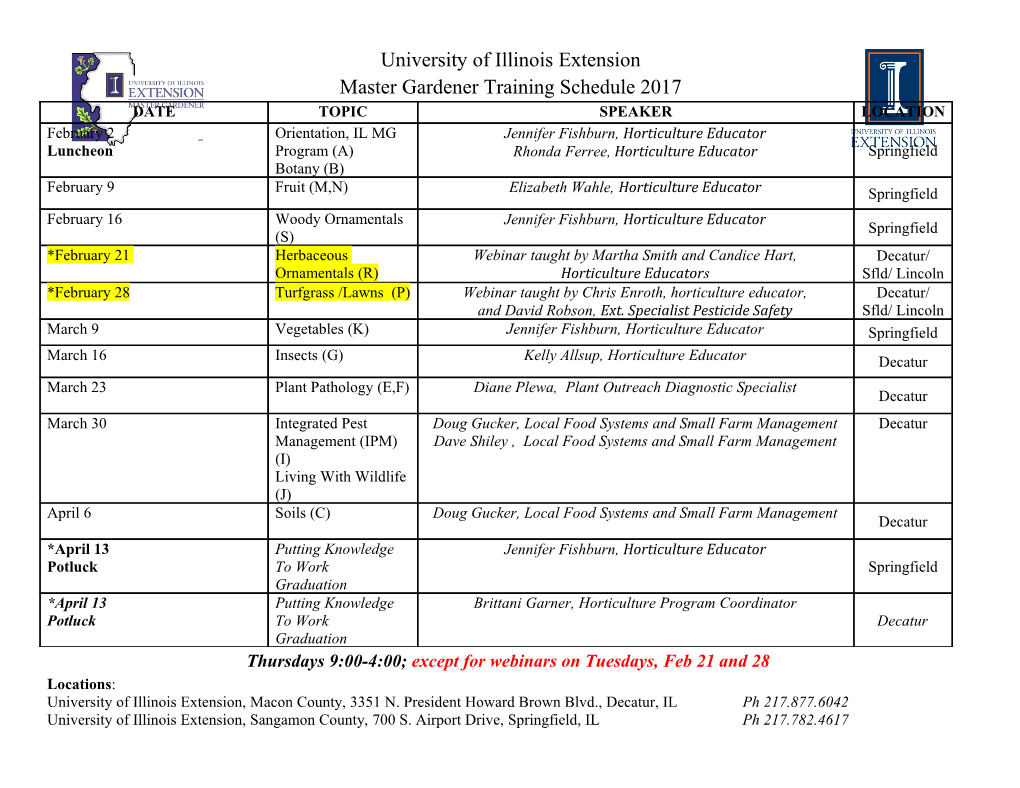
Pacific Earthquake Engineering PacificPacificPacificPacificPacific EarthquakeEarthquakeEarthquakeEarthquake Earthquake EngineeringEngineeringEngineeringEngineering Engineering Research Center ResearchResearchResearchResearchResearch CenterCenterCenterCenter Center Modeling Soil Liquefaction Hazards for Performance-Based Earthquake Engineering Steven L. Kramer University of Washington Ahmed-W. Elgamal University of California, San Diego A report on research conducted under grant no. EEC-9701568 from the National Science Foundation PEER 2001/12 PEERPEER 2001/06 2001/13 PEERSEPTEMBER PEER2001/062002/03 2001/07 2001 februaryAPRIL 1999 2001 aUGUSTPEERAPRILPEER 2001/09AUG. 2001/10 1999 2002 2001 Septemberdec. 2001 2001 Modeling Soil Liquefaction Hazards for Performance-Based Earthquake Engineering Steven L. Kramer Professor Department of Civil and Environmental Engineering University of Washington and Ahmed-W. Elgamal Professor Department of Structural Engineering University of California, San Diego A report on research conducted under grant no. EEC-9701568 from the National Science Foundation PEER Report 2001/13 Pacific Earthquake Engineering Research Center College of Engineering University of California, Berkeley February 2001 EXECUTIVE SUMMARY Introduction The performance of structures such as buildings and bridges during earthquakes is strongly influenced by the performance of the soils that support them. Local soil conditions can influence structural performance in two primary ways — by influencing the ground motions that excite the structure and by imposing additional deformations on the structure through ground failure. The first widespread observations of damage attributed to liquefaction were made in the 1964 Niigata, Japan, and 1964 Alaska earthquakes. In numerous earthquakes since, liquefaction has been deemed responsible for significant damage to buildings and bridges. Liquefaction has been studied extensively over the past 35 years, and substantial advances have been made in understanding the development and effects of this phenomenon. These advances have led to a series of practical procedures for evaluating the potential for occurrence and for estimating its effects. These procedures, however, are almost entirely empirical in nature and, as such, are difficult to apply to the problem of performance prediction for individual structures. The Pacific Earthquake Engineering Research (PEER) Center is committed to the development of methods and procedures for performance-based earthquake engineering (PBEE). The use of PBEE requires the ability to predict a nearly continuous spectrum of performance states for individual structures. This requirement implies the need for tools and procedures for evaluation of the entire process of liquefaction, from initiation to effects. Recent advances in the understanding of liquefaction-related phenomena offer the promise of improved analytical predictions of the initiation and effects of soil liquefaction. Background Soil liquefaction is a complicated phenomenon that can manifest itself in several different ways in the field. When high porewater pressures are generated in a substantially thick soil layer that is relatively near the ground surface, the upward flowing porewater may carry sand particles up to the ground surface where they are deposited in a generally conical pile called a sand boil. While sand boils represent the most common evidence of subsurface soil liquefaction, they are not damaging by themselves. Liquefaction can, however, produce significant soil deformations, both horizontal and vertical, that can cause significant damage to a variety of structures. iii Soil liquefaction has attracted considerable attention from geotechnical engineering researchers over the past 35 years. Liquefaction research has been undertaken from several different perspectives, which has led to some ambiguity and inconsistency in the terminology used to describe various liquefaction-related phenomena. For example, liquefaction was viewed by one group of researchers to correspond to the condition at which the effective stress reaches (temporarily) a value of zero, while another group considered liquefaction to have occurred when the soil deforms to large strains under constant shearing resistance. The first phenomenon is now referred to as cyclic mobility and the second as flow liquefaction. In the field, significant lateral deformations can be caused by either of these phenomena. The deformations produced by flow liquefaction are usually referred to as flow slides, and those produced by cyclic mobility as lateral spreads, but it is frequently impossible to distinguish between the two in the field. Further complicating matters is the fact that some flow slides begin as lateral spreads, so that the final deformations reflect both phenomena. To date, most research into liquefaction hazards has concentrated on the question of liquefaction potential, i.e., whether or not liquefaction will occur. The influence of liquefaction on the performance of structures, however, depends on the effects of liquefaction. While estimation of liquefaction effects has been improved by development of empirical procedures, the uncertainty involved in predicting these effects is still extremely high. More reliable prediction of structural performance requires more accurate prediction of liquefaction effects. A significant portion of current and planned PEER research, in both the core and lifelines programs, is directed toward improved prediction of the effects of soil liquefaction. This research includes, and will eventually rely upon, the development of accurate, reliable, and practical models for soil liquefaction. Contemporary Issues in Liquefaction Modeling The geotechnical engineering profession’s understanding of the mechanics of soil liquefaction has improved greatly in recent years. The process by which excess porewater pressure increases and stiffness decreases at both low and high strain levels is well understood conceptually, although the experimental database for high strain behavior is quite limited. This improved understanding has allowed the development of constitutive models that capture important aspects of soil behavior that were not included in previous liquefaction models. Several PEER iv researchers have made significant advances in this area, and continue to address contemporary issues in liquefaction modeling. The development of models capable of predicting the effects of liquefaction requires that several issues be addressed. First, the model should be capable of accounting for phase transformation behavior. Second, the model should consider the effects of pore-pressure (and void-ratio) redistribution. Third, the model should account for the residual strength of the soil. Fluctuations in the porewater pressure generated during laboratory tests on liquefiable soils have been observed for many years, but the phenomenon responsible for their occurrence has become well understood only relatively recently. In the early stages of cyclic loading, whether in the laboratory or in the field, liquefiable soils exhibit contractive behavior that causes porewater pressure to increase and effective stress to decrease. At high stress ratios (ratio of shear stress to effective normal stress), however, the soil can exhibit dilative behavior. Dilative behavior leads to reduced porewater pressure and increased effective stress. The condition at which the behavior changes from contractive to dilative, i.e., the phase transformation state, occurs at a particular stress ratio; when plotted graphically in stress path space, this stress ratio is referred to as the “phase transformation line.” The dilation associated with phase transformation behavior leads to stiffening and strengthening of a liquefied soil with increasing strain level. Therefore, phase transformation behavior plays a key role in determining the level of deformations, both cyclic and permanent, that can develop in a liquefied soil deposit. Because performance is so closely related to deformations, consideration of phase transformation behavior is a crucial part of liquefaction modeling for performance-based earthquake engineering. As excess porewater pressures develop in a liquefiable soil deposit, the resulting hydraulic gradients cause the porewater to flow from regions of high hydraulic head to regions of low hydraulic head both during and after earthquake shaking. Frequently, this flow occurs in an upward direction and can, when a sufficient volume of water moves upward fast enough, lead to the ejection of sand and formation of sand boils at the ground surface. At some sites, however, the presence of low-permeability layers of soil impedes the flow of water. In such cases, porewater pressures increase in the vicinity of these layers, often for some period of time following the end of earthquake shaking. As the porewater pressure increases, the effective stress decreases, and the soil rebounds to a higher void ratio. At the higher void ratio, the v residual strength of the soil decreases (often significantly because residual strength is sensitive to void ratio), which can lead to the type of flow sliding that has frequently been observed following earthquakes in the past. Reliable prediction of the performance of structures in areas underlain by liquefiable soil requires that this important mechanism be considered in liquefaction hazard analysis; hence, liquefaction models should be capable of accounting for this type of behavior. When a soil
Details
-
File Typepdf
-
Upload Time-
-
Content LanguagesEnglish
-
Upload UserAnonymous/Not logged-in
-
File Pages186 Page
-
File Size-