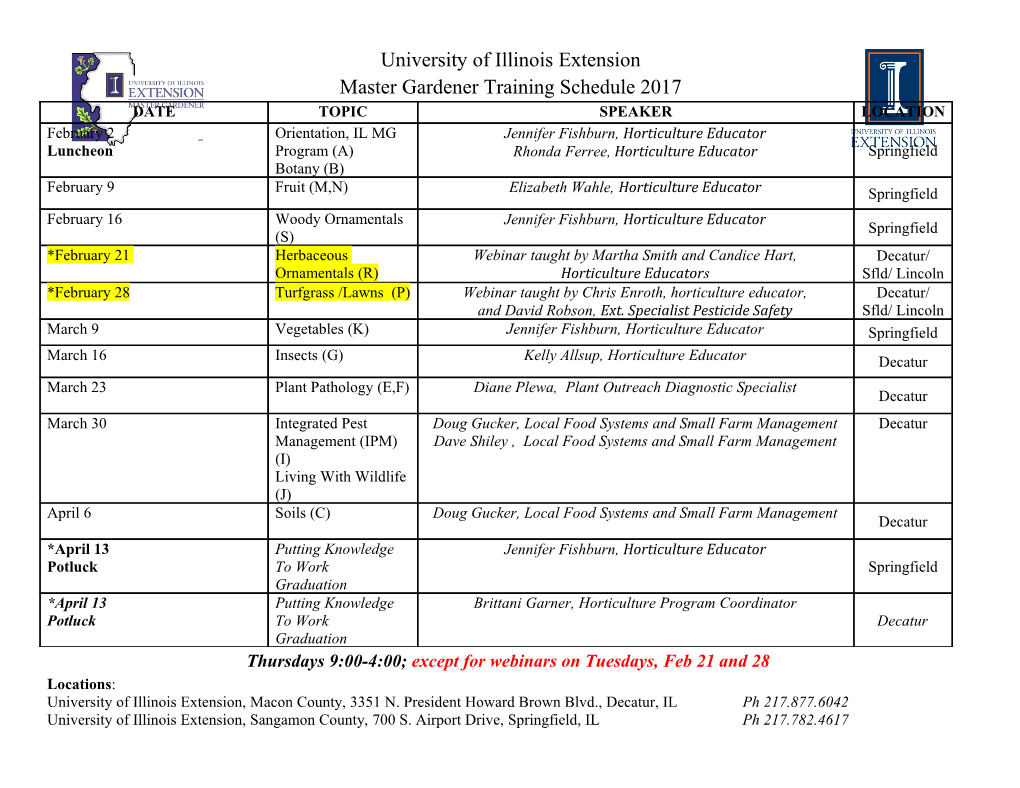
Functions and mechanisms of non-histone protein acetylation Narita, Takeo; Weinert, Brian T; Choudhary, Chunaram Published in: Nature Reviews. Molecular Cell Biology DOI: 10.1038/s41580-018-0081-3 Publication date: 2019 Document version Peer reviewed version Citation for published version (APA): Narita, T., Weinert, B. T., & Choudhary, C. (2019). Functions and mechanisms of non-histone protein acetylation. Nature Reviews. Molecular Cell Biology, 20, 156-174. https://doi.org/10.1038/s41580-018-0081-3 Download date: 26. sep.. 2021 Functions and mechanisms of non-histone protein acetylation Takeo Narita, Brian T. Weinert, Chunaram Choudhary* The Novo Nordisk Foundation Center for Protein Research, Faculty of Health Sciences, University of Copenhagen, Blegdamsvej 3, 2200 Copenhagen, Denmark *Correspondence should be addressed to: CC, [email protected] Abstract | N-ε-lysine acetylation was discovered more than half a century ago as a posttranslational modification of histones and has been extensively studied in the context of transcription regulation. In the past decade, proteomic analyses have revealed that non-histone proteins are frequently acetylated and constitute a major portion of the acetylome in mammalian cells. Indeed, non-histone protein acetylation is involved in key cellular processes relevant to physiology and disease, such as gene transcription, DNA damage repair, cell division, signal transduction, protein folding, autophagy and metabolism. Acetylation affects protein functions through diverse mechanisms, including by regulating protein stability, enzymatic activity, subcellular localization, cross-talk with other posttranslational modifications, and by controlling protein–protein and protein–DNA interactions. In this Review, we discuss recent progress in our understanding of the scope, functional diversity and mechanisms of non-histone protein acetylation. Introduction Precise control of protein function is essential for the organization and function of biological systems. Among different regulatory processes, reversible posttranslational modifications (PTMs) provide an elegant mechanism to govern protein function. A key advantage of PTMs is that they can be dynamically regulated at a much faster rate and with a lower energy cost compared with protein turnover. Eukaryotic proteomes contain hundreds of different types of PTMs; however, only a small number of them, such as phosphorylation, glycosylation, methylation, acetylation, ubiquitylation, and sumoylation have been studied extensively. 1 Although the physiological importance of PTMs has been known for more than half a century, the widespread occurrence of PTMs only started to become clear in the first years of the 21st century, when advances in high-resolution mass spectrometry enabled detection of thousands of low- abundance PTM sites1. It is also increasingly appreciated that combinations of PTMs can generate distinct protein isoforms with varying functions, which vastly expand the functional diversity of mammalian proteomes2. Lysine acetylation is an evolutionarily conserved PTM, occurring in both prokaryotes and eukaryotes. Acetylation was first discovered on histones by Vincent Allfrey and colleagues in 19643. Subsequently, acetylation was found on high mobility group (HMG) proteins4, which are chromatin-binding non-histone proteins, and on tubulin5. In the mid to late 1990s, acetylation of the transcription factor p53 was discovered, the first mammalian histone acetyltransferases (HATs) and histone deacetylases (HDACs) were identified, the bromodomain was identified as an acetyl-lysine reader domain, and potent deacetylase inhibitors were discovered (reviewed in6). These groundbreaking discoveries set the stage for the field of non-histone protein acetylation. We wish to clarify that the term ‘acetylation’ can encompass other types of protein acetylation, such as N-terminal protein acetylation and O-linked acetylation of serine and threonine. Unless otherwise specified, in this Review ‘acetylation’ refers only to N-ε-lysine acetylation. Over the past decade, advances in mass-spectrometry-based proteomics have vastly expanded the catalogue of endogenously acetylated proteins, provided an unbiased view of the acetylome, and revealed new insights into the scope and regulation of non-histone protein acetylation. In appreciation of the extent of non-histone acetylation, HATs and HDACs were renamed to lysine acetyltransferases (KATs) and lysine deacetylases (KDACs), respectively. The identification of thousands of acetylation sites has spurred great interest in wide range of biomedical research communities, and non-histone protein acetylation has been implicated in all major biological processes (see below). In this review, we provide an overview of the expanding landscape of non-histone protein acetylation. We discuss the subcellular, compartment-specific generation of the acetyl-group 2 donor acetyl-CoA, enzymatic regulation of acetylation, and the emerging non-enzymatic mechanisms of acetylation. The major focus is on acetylation, however, related lysine acylations are also briefly discussed. Although it is challenging to comprehensively review this rapidly growing field, we discuss a plethora of functionally characterized acetylation sites on non-histone proteins to illustrate the functional diversity and mechanistic principles of acetylation. We also briefly describe the disease and therapeutic relevance of acetylation, and conclude with discussing key open questions and future perspectives. Because histone acylation was extensively covered in a recent review7, it will not be covered here. The scope of non-histone acetylation Until the beginning of the 21st century, acetylation was mostly identified on individual proteins using conventional approaches, such as in vitro acetyltransferase assays with radioisotope labeled acetyl-CoA or using acetyl-lysine antibodies. In 2006, a combination of acetylated peptide immunoaffinity-enrichment and high-resolution mass spectrometry enabled the identification of hundreds of acetylation sites8. Subsequently, the identification of thousands of acetylation sites from human cell lines was reported9. These unbiased proteomic analyses showed that acetylation was a surprisingly common modification of proteins in diverse cellular compartments. Many ensuing studies firmly established that, in addition to histones, acetylation occurs on tens of thousands of non-histone proteins in evolutionarily diverse organisms10. In recent years mass- spectrometry-based studies have quantified the relative changes at thousands of acetylation sites in response to genetic, chemical, and metabolic perturbations, and provided insights into the dynamic regulation of lysine acetylation11-15. Regulation of acetylation Acetylation is generated by KAT-catalyzed transfer of an acetyl group from acetyl-CoA to the - amino side chain of lysine, and is reversed by KDACs (Fig. 1a). Recent studies show that acetylation also occurs through non-enzymatic mechanisms and is affected by the availability of acetyl-CoA (BOX 1). Regulation of acetyl-CoA synthesis 3 Acetyl-CoA is a key metabolite with essential cellular functions, such as energy generation in the mitochondria and biosynthesis of lipids in the cytoplasm. Because acetyl-CoA is membrane- impermeable, mitochondrial and non-mitochondrial pools of acetyl-CoA are generated independently (Fig. 1b). Depending on the nutrient source, mitochondrial acetyl-CoA is generated by the pyruvate dehydrogenase complex (PDC), by beta-oxidation of fatty acids, or through amino acid metabolism. The non-mitochondrial pool of acetyl-CoA is generated in the cytoplasm and nucleus by ATP-citrate lyase (ACLY; also known as ATP-citrate synthase) and acyl-CoA synthetase short-chain family member 2 (ACSS2), as well as by the nuclear PDC. Acetyl-CoA can freely diffuse between the cytoplasm and nucleus through the nuclear pores. Acetylation is directly linked to acetyl-CoA levels, and cell-compartment-specific generation of acetyl-CoA can locally fuel acetylation. For example, nuclear ACLY, ACSS2 and PDC are reported to regulate histone acetylation and gene transcription through localized production of acetyl-CoA16. In yeast, depletion of mitochondrial acetyl-CoA only ablates acetylation of mitochondrial proteins, without affecting acetylation of nuclear proteins17. In mice, deletion of both acetyl-CoA carboxylase 1 (ACC1) and ACC2, which convert cytoplasmic acetyl-CoA to malonyl-CoA (Fig. 1b), results in increased protein acetylation18, likely through an increase in the levels of acetyl-CoA. Fluctuations in acetyl-CoA levels by genetic and dietary manipulations correlate with changes in acetylation levels, further indicating that acetyl-CoA is a rate-limiting factor for many acetylation events (reviewed in13). Lysine acetyltransferases The exact number of bona fide KATs in the human proteome is unclear. Among the reported KATs, thirteen are well-characterized (‘canonical’) and a majority of them are classified into three families: GCN5, p300 and MYST19 (Fig. 1c). The remaining KATs: alpha-tubulin N-acetyltransferase 1 (ATAT1; also known as TAT1), ESCO1 and ESCO2, and histone acetyltransferase 1 (HAT1), are relatively dissimilar to each other. With the exception of TAT1, all of the canonical KATs are primarily localized in the nucleus and acetylate histones and non-histone proteins. Compared with protein kinases, much less is known about the substrate specificities of KATs. The substrate specificity of KATs is thought
Details
-
File Typepdf
-
Upload Time-
-
Content LanguagesEnglish
-
Upload UserAnonymous/Not logged-in
-
File Pages128 Page
-
File Size-