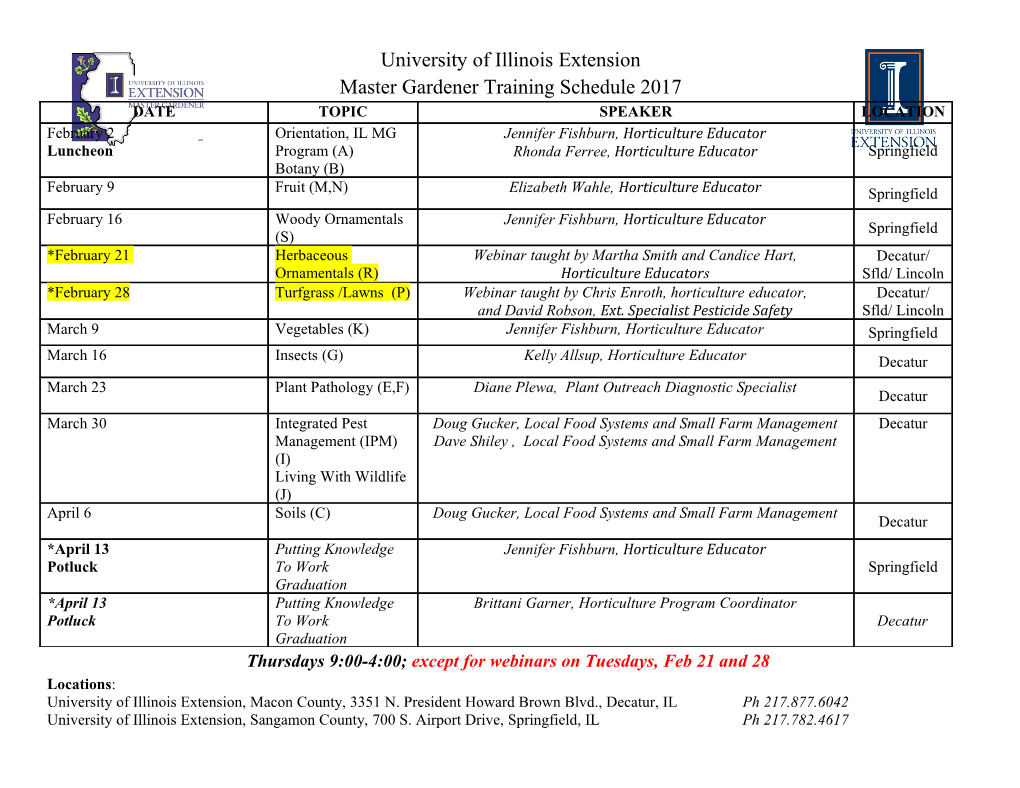
A Compact Artificial Gill using a Hemoglobin Solution as a Thermo-responsive Oxygen Carrier On-line Number 349 Kenichi Nagase, Fukashi Kohori, and Kiyotaka Sakai Department of Chemical Engineering, Waseda University, 3-4-1 Okubo, Shinjuku-ku, Tokyo 169-8555, Japan E-mail: [email protected] ABSTRACT A compact artificial gill was developed using a concentrated hemoglobin solution containing inositol hexaphosphate (IHP) as the oxygen carrier solution. Oxygen dissolved in seawater is first taken up from water to the oxygen carrier solution at 293 K. The oxygen carrier solution is then heated and the oxygen is released from the oxygen carrier solution to expired air at 310 K. The enhancement factors of oxygen carrier solution that indicates its performance were approximately 3 for the oxygen uptake and 16 for the oxygen release, respectively. The scaling up for a human at rest was estimated. The required membrane surface area and seawater flow rates were 63.8 m2 and 0.00653 m3/s, respectively. These values indicate the feasibility of a compact and portable artificial gill for human underwater activity. KEYWORDS Artificial gill, Oxygen transfer, Hemoglobin, Facilitated transport INTRODUCTION An artificial gill is a device for the uptake of oxygen from water to air through a gas permeable membrane, driven by an oxygen partial pressure difference between the water and air. It enables humans to breathe underwater, thereby extending the time that can be spent underwater, whether for scuba diving, sea rescue, sea exploration, or in a sea-bed city. It could help to achieve the dream of swimming like fish and living in the sea. In the earliest studies, Ayres (1966) indicated the possibility of an artificial gill using silicone rubber membrane. Bodell (1965) has reported a gas transfer apparatus with coils of capillary tubes made of silicone rubber that was able to sustain a rat for 25 h. Paganelli et al. (1967) designed an artificial gill consisting of a chamber covered on one end with a gas-permeable membrane. Yang and Cussler (1989) developed a hollow fiber membrane module that was able to sustain a dog. Matsuda and Sakai (1999) compared the gas transfer mechanism of a fish gill with that of an artificial gill consisting of hollow fiber membrane, and proposed an optimum structure for an artificial gill. However, these artificial gills are not yet practical, because the oxygen transfer rate from water is not adequate, and the oxygen partial pressure provided in the air is too low for human respiration. On the other hand, an artificial gill using oxygen absorbent was developed to enhance the oxygen transfer. Bonaventure and Bonaventura (1982) increased oxygen transfer rate from water to air using an oxygen absorbent containing hemoglobin in a polyurethane matrix. However, a very large apparatus was required to release oxygen from hemoglobin, because the oxygen affinity was controlled by pH. We have developed several artificial gill systems mimicking fish gills and using an oxygen carrier solution. These artificial gills consist of two hollow fiber membrane modules and an oxygen carrier solution. One module is for oxygen uptake from water to the oxygen carrier solution, and the other is for oxygen release from the oxygen carrier solution to air. Several oxygen carrier solutions have been 1 tested for an artificial gill. First, perfluorocarbon (PFC) was used as the oxygen carrier solution. PFC is a general name for a family of highly fluorinated organic compounds, which are chemically and biologically inert. Some of these have been used as blood substitutes because of their unusual ability to dissolve oxygen and other respiratory gases (Riess and Blanc 1982, Lowe 2001). Haramoto et al. (1994) have reported an artificial gill using perfluorooctylbromide (PFOB), which is one of the PFCs, as an oxygen carrier solution. This artificial gill greatly increases the oxygen transfer rate from water to PFOB, but not from PFOB to air. Matsuda et al. (2001) developed a novel artificial gill using a red blood cell (RBC) suspension as a thermo-responsive oxygen carrier solution. Dissolved oxygen is taken up into the Figure 1. Artificial gill using a oxygen carrier solution, which is then concentrated hemoglobin solution with IHP heated to release oxygen into the air. This new system greatly increases the oxygen transfer rate, however the excessive oxygen affinity of hemoglobin must also be optimized for an artificial gill. Nagase et al. (2002) optimized the oxygen affinity of hemoglobin in the artificial gill by adding inositol hexaphosphate (IHP) as an allosteric effector at varying pH. The use of this oxygen carrier solution may enhance oxygen transfer from water to air. In the present study, we designed a compact artificial gill using concentrated hemoglobin solution containing IHP as the oxygen carrier solution (Figure 1). The enhancement factors of the oxygen carrier solution that indicates its performance were obtained from the oxygen uptake rate and oxygen release rate to estimate the oxygen transfer rate through the membrane oxygenator. The scaling up for a human at rest was estimated using the enhancement factors and the correlations of a membrane oxygenator. MATERIALS AND METHODS Preparation of oxygen carrier solution Bovine red blood cells were hemolyzed by adding a large quantity of pure water, and the RBC membranes were removed using a plasma separator (Cascadeflo, Asahi Medical, Tokyo, Japan). This solution was sterilized using a sterile filtration membrane (Milex-GP50, pore size: 0.22 µm; Millipore, Bedford, MA), and concentrated to 5.43 µmol/cm3 using a high-performance dialyzer (AM-SD-150H, Asahi Medical). Inositol hexaphosphate was added to the purified hemoglobin solution at a molar ratio of hemoglobin to IHP 1:5, and pH was adjusted to 6.9 using potassium phosphate (WAKO, Osaka, Japan) and sodium phosphate dibasic (WAKO). 2 Measurement of enhancement factors of oxygen carrier solution The enhancement factors of the oxygen carrier solution were measured using a miniaturized hollow fiber membrane module. The hollow fiber was made of porous polypropylene with an inner diameter of 0.25 mm, an outer diameter of 0.3 mm, and a membrane thickness of 25 µm; only the outside was coated with silicone. The number of hollow fibers was 200, the effective length of the hollow fibers was 100 mm, and membrane surface area was 0.0188 m2. This module was used in two systems, oxygen uptake (seawater – oxygen carrier solution) and oxygen release (oxygen carrier solution – expired air). The oxygen uptake rate was measured using the apparatus shown in Figure 2. The oxygen carrier solution was made to flow inside the hollow fibers at a flow rate of 0.083 cm3/s by a syringe pump. Pure water instead of seawater was made to Figure 2. Schematic of apparatus for measuring flow outside the hollow fibers at a rate of enhancement factor in oxygen uptake 1.33 cm3/s by gravity. Temperature was maintained at a constant 293 K. The oxygen concentration in water and oxygen carrier solution at module inlet and outlet was measured using a dissolved oxygen meter (TOX-90i, Toko Chemical Laboratory, Tokyo). The oxygen uptake rate N (mol/s) was obtained from the following equations N = QW {α(pW ,in − pW ,out )} (1) N = QC {α(pC ,out − pC ,in )+ β (SC ,out − SC ,in )} (2) where Q (m3/s) is the flow rate, p (Pa) is the oxygen partial pressure, S (-) is the oxygen saturation of hemoglobin, α (mol m-3 Pa-1) the physical solubility of oxygen, and β (mol/m3) the amount of oxygen chemically bonded with a unit volume of the oxygen carrier solution. Subscript W and C indicate water and oxygen carrier solution, respectively, and subscript in and out indicate the module inlet and outlet, respectively. The oxygen release rate was measured using the apparatus shown in Figure 3. The oxygen carrier solution was made to flow outside the hollow fibers at a flow rate of 0.333 cm3/s by a syringe pump. Expired air (oxygen partial pressure: 15.4 kPa) was made to flow inside the hollow fibers at a flow rate of 0.167 cm3/s. Temperature was maintained at 310 K. The oxygen concentration in water and oxygen Figure 3. Schematic of apparatus for measuring enhancement factor in oxygen release carrier solution at module inlet and outlet 3 was measured using a dissolved oxygen meter (TOX-90i). The oxygen release rate was obtained from the difference of oxygen concentration between module inlet and outlet. N = QC {α(pC ,in − pC ,out )+ β (SC ,in − SC ,out )} (3) The overall mass transfer coefficients for oxygen uptake and oxygen release were obtained by following equation N K = (4) Aα∆plm where ∆plm (Pa) is the logarithmic mean difference of oxygen partial pressure. The enhancement factors of oxygen carrier solution are obtained from the ratio of mass transfer coefficient of oxygen carrier solution to that of water in place of oxygen carrier solution. This value indicates the performance of oxygen carrier solution (Nagase et al., 2003). k E = C (5) k L where kC (m/s) is the mass transfer coefficient of oxygen carrier solution, kL (m/s) is the mass transfer coefficient of water in place of oxygen carrier solution. These values are obtained by deducting membrane- and water-side resistances from the overall mass transfer resistance, which is the reciprocal of the overall mass transfer coefficient. 4 RESULTS AND DISCUSSION Changes in oxygen partial pressure in oxygen carrier solution with temperature The oxygen partial pressure of the oxygen carrier solution was measured before and after heating (from 293 K to 310 K) in the closed system. Figure 4 shows the relationship of oxygen partial pressure of oxygen carrier solution before and after heating from 293 K to 310 K.
Details
-
File Typepdf
-
Upload Time-
-
Content LanguagesEnglish
-
Upload UserAnonymous/Not logged-in
-
File Pages8 Page
-
File Size-