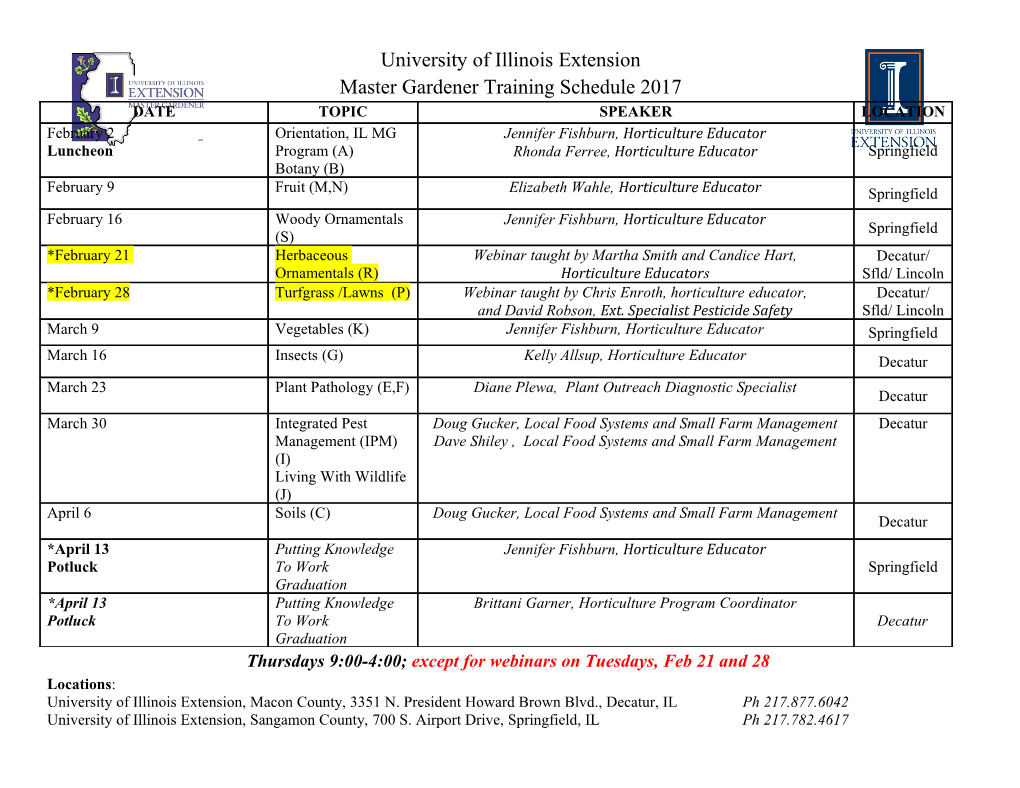
EPSC Abstracts Vol. 14, EPSC2020-515, 2020 https://doi.org/10.5194/epsc2020-515 Europlanet Science Congress 2020 © Author(s) 2021. This work is distributed under the Creative Commons Attribution 4.0 License. Physical Evolution Model for Jupiter-Family Comets and Centaurs Luke Dones1 and Maria Womack2 1Southwest Research Institute, Boulder, Colorado, USA ([email protected]) 2Florida Space Institute and Department of Physics, University of Central Florida, Orlando, Florida, USA ([email protected]) Centaurs – planet-crossing bodies in the region of the giant planets that mainly originate in the Kuiper Belt/Scattered Disk [1, 2] – are thought to be the primary impactors on the giant planets and their satellites [3-8]. As part of an effort to interpret the cratering records of the saturnian satellites, we are developing a dynamical-physical model for the size distribution of potential impactors on the moons. Most models of the orbital distribution of "observable" comets[1] assume that the size of the nucleus does not change with time. These models treat physical evolution only by assuming a lifetime, after which comets are considered inactive or "faded". These models do not specify a fading mechanism, but assume an expression for the probability that a comet remains active after some amount of time [10-14]. Fading can result from loss of all volatiles, formation of a nonvolatile mantle on the surface of the nucleus, or splitting [15, 16]. A model of the erosion of 67P/Churyumov-Gerasimenko and 46P/Wirtanen due to sublimation of water ice throughout their orbital evolution estimates that 67P’s nucleus has shrunk from a radius of 2.5 km to 2 km, while 46P’s has decreased from 1 km to 0.6 km [17]. This calculation assumes that 10% of the nucleus is active and that its density is 500 kg/m3. These estimates are uncertain because comets follow chaotic orbits, but in general, erosion has a bigger effect on smaller nuclei. Some comets are active well beyond the water-ice sublimation zone within 3 au. Eighteen active Centaurs are currently known [18, 19]. 29P/Schwassmann-Wachmann, which follows a near-circular orbit at 6 au, is a copious source of dust and CO [20-22] and undergoes significant dust outbursts 7 or more times a year [23]. 174P/Echeclus underwent an outburst 13 au from the Sun that released ≈ 300 kg/s of dust for about two months [24], a rate comparable to the 530 kg/s of dust released by 67P at its peak near 1.3 au [25]. Echeclus also underwent several more outbursts near perihelion (≈6 au) with CO outgassing at ≈ 10% the rate of 29P at the same heliocentric distance and dust mass loss rates of 10 - 700 kg/s [20]. 2060 Chiron is another Centaur that is sporadically active in gas and dust, consistent with a more depleted state, like Echeclus [20]. Di Sisto et al. (2009) constructed a model of the orbital distributions of Jupiter-family comets (JFCs) that incorporated planetary perturbations, nongravitational forces, sublimation, and splitting. They considered nuclei with initial radii of 10, 5, and 1 km [26]. Di Sisto et al. found that 5- and 10-km comets usually evolved onto Centaur orbits, while 1-km comets were most likely to shrink below 100 m. Inspired by their work, we are developing a model for the dynamical-physical evolution of JFCs and Centaurs. We will use the orbital distribution found by Nesvorny et al. as our baseline model [14, 27]. We will first focus on modeling the evolution of the size distribution of JFCs. The model will eventually account for mass loss by both JFCs and Centaurs, with activity driven by H2O, CO, or other volatiles. The fraction of the nucleus that is active will be allowed to vary with size, since smaller nuclei are typically more active [28-30]. We will then implement a model for cometary splitting with these inputs: the frequency of splitting as a function of perihelion distance; the fraction of the comet’s mass released as fragments; the size distribution of the fragments; and the velocity imparted to the fragments by the splitting event. We will present preliminary results of our simulations. We thank Raphael Marschall for discussions and the Cassini Data Analysis Program for support. References [1] Volk, K.; Malhotra, R. ApJ 687, 714–725, 2008. [2] Di Sisto, R. P.; Rossignoli, N. L. CMDA, in press (arXiv:2006.09657), 2020. [3] Zahnle, K.; Dones, L.; Levison, H. F. Icarus 136, 202–222, 1998. [4] Zahnle, K.; Schenk, P.; Levison, H.; Dones, L. Icarus 163, 263–289, 2003. [5] Di Sisto, R. P.; Zanardi, M. A&A 553, id. A79, 2013. [6] Di Sisto, R. P.; Zanardi, M. Icarus 264, 90–101, 2016. [7] Rossignoli, N. L.; Di Sisto, R. P.; Zanardi, M.; Dugaro, A. A&A 627, id. A12, 2019. [8] Wong, E. W.; Brasser, R.; Werner, S. C. EPSL 506, 407–416, 2019. [9] Quinn, T.; Tremaine, S.; Duncan, M. ApJ 355, 667–679, 1990. [10] Oort, J. H. BAN 11, 91–110, 1950. [11] Levison, H. F.; Duncan, M. J. Icarus 127, 13–32, 1997. [12] Wiegert, P.; Tremaine, S. Icarus 137, 84–121, 1999. [13] Brasser, R.; Wang, J.-H. A&A 573, id. A102, 2015. [14] Nesvorný, D. et al. ApJ 845, id. 27, 2017. [15] Weissman, P. R.; Bottke, W. F., Jr.; Levison, H. F. In Asteroids III, Univ. Arizona Press, pp. 669–686, 2002. [16] Jewitt, D. C. In Comets II, Univ. Arizona Press, pp. 659–676, 2004. [17] Groussin, O. et al. MNRAS 376, 1399–1406, 2007. [18] Jewitt, D. AJ 137, 4296–4312, 2009. [19] Chandler, C. O. et al. ApJLett 892, id. L38, 2020. [20] Womack, M.; Sarid, G.; Wierzchos, K. PASP 129, 031001, 2017. [21] Sarid, G. et al. ApJLett 883, id. L25, 2019. [22] Wierzchos, K.; Womack, M. AJ 159, id. 136, 2020. [23] Trigo-Rodríguez, J. M. et al. MNRAS 409, 1682–1690, 2010. [24] Bauer, J. M. et al. PASP 120, 393–404, 2008. [25] Marschall, R. et al. Frontiers in Physics, doi: 10.3389/fphy.2020.00227 (arXiv:2005.13700), 2020. [26] Di Sisto, R. P.; Fernandez, J. A.; Brunini, A. Icarus 203, 140–154, 2009. [27] Nesvorný, D. et al. AJ 158, id. 132, 2019. [28] A’Hearn, M. F. et al. Science 332, 1396–1400, 2011. [29] Tancredi, G.; Fernandez, J. A.; Rickman, H.; Licandro, J. Icarus 182, 527–549, 2006. [30] Schleicher, D. G.; Knight, Matthew M. AJ 152, id. 89, 2016. [1] The simplest model used to compare simulated and observed orbital distributions assumes that comets that pass perihelion within a fixed distance from the Sun, such as 2.5 au, are "discovered" [9]. Powered by TCPDF (www.tcpdf.org).
Details
-
File Typepdf
-
Upload Time-
-
Content LanguagesEnglish
-
Upload UserAnonymous/Not logged-in
-
File Pages3 Page
-
File Size-