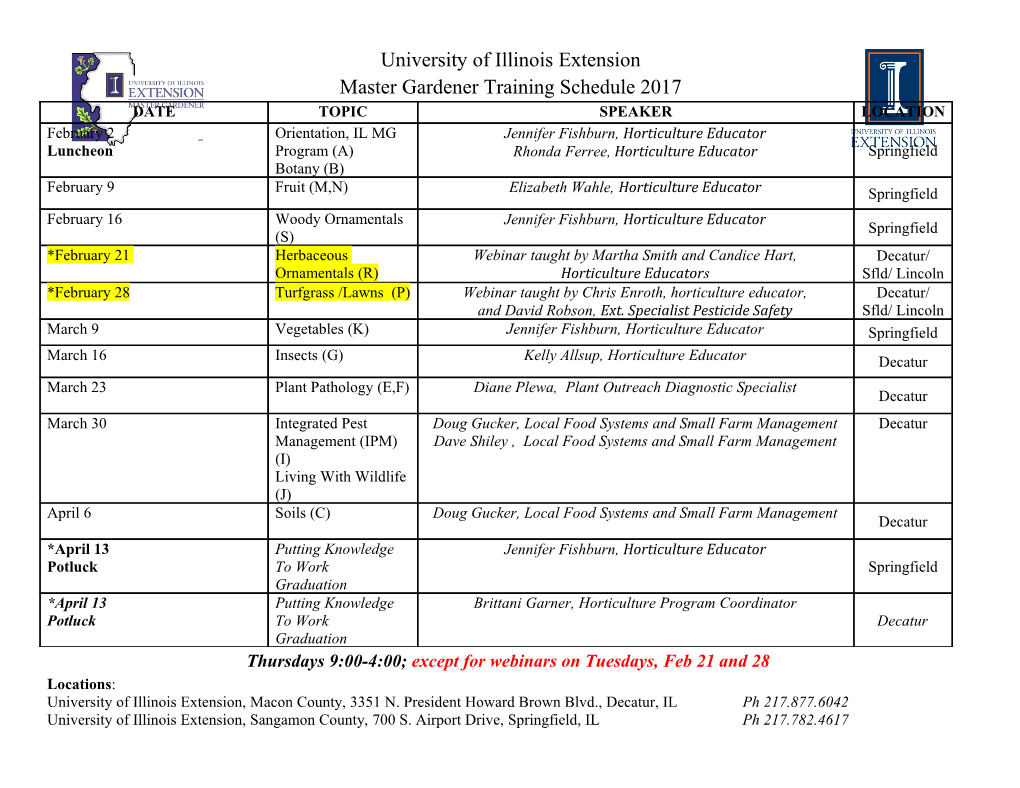
4570 CARBON 48 (2010) 4556– 4577 The large-scale production of graphene flakes using magnetically-enhanced arc discharge between carbon electrodes I. Levchenko a,b, O. Volotskova c, A. Shashurin c,d, Y. Raitses e, K. Ostrikov a,b,, M. Keidar c a CSIRO Materials Science and Engineering, P.O. Box 218, Lindfield NSW 2070, Australia b School of Physics, The University of Sydney, Sydney NSW 2006, Australia c Department of Mechanical and Aerospace Engineering, The George Washington University, Washington, DC 20052, USA d Applied Plasma Science LLC, Marlowe Place, Oak Park, MI 25930, USA e Princeton Plasma Physics Laboratory, Princeton, NJ 08543, USA ARTICLE INFO ABSTRACT Article history: A novel approach to large-scale production of high-quality graphene flakes in magneti- Received 28 May 2010 cally-enhanced arc discharges between carbon electrodes is reported. A non-uniform mag- Accepted 29 July 2010 netic field is used to control the growth and deposition zones, where the Y–Ni catalyst Available online 7 August 2010 experiences a transition to the ferromagnetic state, which in turn leads to the graphene deposition in a collection area. The quality of the produced material is characterized by the SEM, TEM, AFM, and Raman techniques. The proposed growth mechanism is supported by the nucleation and growth model. Ó 2010 Elsevier Ltd. All rights reserved. Graphene and graphene flakes (GFs) are very promising for Here we report on a novel method for the large-scale pro- various carbon-based nanodevices (single-electron transis- duction of graphene flakes in an arc discharge, enhanced with tors, supercapacitors, sensors, etc.) [1,2]. GFs also demon- a specially shaped magnetic field and a custom-designed cat- strate unique mechanical properties, thus being the most alyst. Using our approach, it is possible to achieve production prominent candidates for the micro- and nano-electrome- yields of graphene flakes which exceed the rates achievable chanical systems [3]. With graphene’s intrinsic breaking by other techniques. strength reaching 42 N mÀ1, it is the strongest material ever The graphene flakes were produced in a high-current arc discovered in the Universe. The production of graphene flakes discharge setup (see scheme and photo in Fig. 1) with carbon usually requires very specific process conditions, namely a anode and cathode installed in the non-magnetic vacuum large influx of carbon material, relatively high temperatures, chamber. The cathode is a rod of 12 mm dia., and the anode and often a specially prepared catalyst. These conditions is a rod of 6 mm dia. with a hole in the centre of 3 mm dia. can be easily maintained in high-density plasmas [4,5]. Re- The hole was filled with a mixture of graphitic carbon and cently, arc discharge plasmas have been successfully used Y–Ni catalyst powders (in 1:4 ratio), with the average particle for the synthesis of graphene flakes [6,7], but large-scale pro- size of 0.04–4 mm. A discharge-enhancing permanent magnet duction of GFs still remains essentially unresolved. The (50 · 50 · 25 mm) was installed in the chamber to create a majority of the surface-based methods (micro-mechanical non-uniform magnetic field of 1.2 kG in the interelectrode exfoliation, epitaxial growth, decomposition of silicon car- gap of about 1 cm. The simulated topography of the magnetic bide, etc.) have not reached the expected yields [1]. Thus, field is shown in Fig. 1. The process was conducted in a he- the arc discharge still remains the most promising method lium gas at a pressure of 500 Torr, which is very effective for for synthesizing high-quality GFs at industrial scales. Given the carbon nanostructure production [8]; some more details the phenomenal success of arc discharges in the synthesis can be found in our recent publications [9]. of large amounts of high-quality CNTs, further development The production of graphene in the catalyst-free arc dis- of the arc discharge systems for the effective large-scale pro- charge process is also possible, but in this case the use of duction of graphene is a highly-topical issue. hydrogen-containing environment is required to terminate dangling bonds with hydrogen atoms and thus prevent the * Corresponding author at: CSIRO Materials Science and Engineering, P.O. Box 218, Lindfield NSW 2070, Australia. Fax: +61 2 9413 7634. E-mail address: [email protected] (K. Ostrikov). 0008-6223/$ - see front matter Ó 2010 Elsevier Ltd. All rights reserved. doi:10.1016/j.carbon.2010.07.055 CARBON 48 (2010) 4556– 4577 4571 where a strong incoming flux of carbon material to the cata- lyst is maintained; outside of the growth zone, the gas tem- perature (and hence the catalyst temperature) decrease sharply below the Curie point. Consequently, the catalyst par- ticles become ferromagnetic, respond to the magnetic field, and eventually the catalyst particles with the attached graph- ene flakes deposit on the magnet surface, in specific graphene collection areas. Thus, in our experiments the specially de- signed catalyst accelerated the nucleation and growth of graphene, and led to graphene deposition onto the magnet surface before any significant overgrowth takes place. The carbon samples were analysed with SEM, TEM, AFM, Fig. 1 – Field emission scanning electron micrograph and Micro-Raman techniques. The Micro-Raman system (FESEM) images (a) and (b), X-ray diffraction (XRD) pattern of was based on an air-cooled argon 200 mW ion laser, holo- carbon fibers annealed at 1400 °C for 2 h (c), transmission graphic optics, spectrometer with a CCD detector (matrix size electron micrograph (TEM) image of carbon fibers with 5 of 1100 · 330 pixels), power 5 mW, wavelength 514 nm. A wt.% cobalt (d, e) and SAED of cobalt particles (inset of series of Raman measurements over both scattering ranges figure e). (first and second order, which allows to study second order peaks) were made. TEM (JEOL JEM-1200EX) and AFM (Asylum Research MFP3D, tapping-mode in air) imaging was used to graphene sheets from rolling into nanotubes [7]. Since hydro- investigate the structural properties of the graphene flakes. gen exhibits high chemical activity in plasma (it can effec- The AFM tip (Olympus AC240TS silicon cantilevers, force con- tively reduce oxides and carbides), such process may lead to stant k =2NmÀ1, resonance frequency f = 70 kHz) was used low controllability of graphene doping with some dopants. in the analysis. The high-resolution SEM analysis was per- This is why we used a catalyzed process in a pure noble gas formed using a Hitachi field emission S-4700-II FE-SEM micro- environment which is highly flexible with respect to any scope (accelerating voltages from 1 to 30 kV). The samples for graphene compositions. TEM analysis were prepared by dispersion of the carbon soot The application of an external magnetic field strongly in- into aqueous 2% (mass/volume) sodium deoxycholate solu- creases both plasma density and temperature [8]. The plasma tion by sonicating in ice bath for 1 h at 1 W/mL of the applied density is increased by the two main effects: first, by mag- power. Then, the sonicated suspension was centrifuged in a netic confinement that restricts the plasma, and second, by Beckman J-2 centrifuge for 2 h in a JA-20 rotor at a speed of magnetizing electrons and thus creating conditions for more 1885 rad/s and a temperature of 10 °C. After that, the samples effective ionization of the neutral gas atoms [10]. Plasma tem- were placed on a gilder grid (T2000-Cu, square, Electron perature, in turn, increases the magnetic field due to stronger Microscopy Science) for further microanalysis. electric field in the magnetized plasma, in contrast to the In Fig. 2 we show SEM micrographs of carbon material col- non-magnetic conditions. This in turn results in the increase lected on the magnet surfaces, TEM image and SAED pattern heating of the nanostructures in the plasma, and strongly in- of graphene flakes. The estimated size of the GFs is approxi- creased flux of ionized material to the nanostructures; as a re- mately 500–2500 nm, typically with up to 10 graphene layers. sult, fast nucleation and growth is ensured due to the The atomic force microscopy clearly revealed the presence of application of an external magnetic field. flake-like structures with the size of around one micron and a The temperature of the anode surface reaches 3500 K [11], fairly uniform height in the 1–5 nm range (Fig. 2e–g). This cor- and thus, effective evaporation of the metal catalyst particles responds to 3–15 graphene layers in different areas of the released from anode is ensured. In the discharge core, tem- flakes. The Raman characterization of the specimens showed perature reaches 5000 K and hence, further evaporation of the occurrence of a weak D-peak at around 1325 cmÀ1, which the metal catalyst to the plasma is supported [12]. Then, the is related to the relatively small amount of defects in sp2 discharge temperature between electrodes falls down below bonds (Fig. 2h). G-peak at 1582 cmÀ1 and highly symmetrical 2000 K, and the metal catalyst re-nucleates and grows to 2D-peak at 2650 cmÀ1 demonstrate the presence of a rela- small catalyst particles (4 nm in average). Further, when the tively small number of graphene layers in the flakes. It should temperature falls below 1500 K, nucleation and growth of be noted that carbon nanotubes were also found in samples the graphene flakes on the small newly-nucleated catalyst collected elsewhere in the chamber. particles starts. The absolute maximum of the graphene flake production To optimize the growth and deposition of graphene flakes, yield in the arc discharge with carbon electrodes can be esti- we used the optimized composition of the two transition met- mated using the measured ablation rate of the graphite an- als, Yttrium (paramagnetic, which easily forms carbides en- ode.
Details
-
File Typepdf
-
Upload Time-
-
Content LanguagesEnglish
-
Upload UserAnonymous/Not logged-in
-
File Pages5 Page
-
File Size-