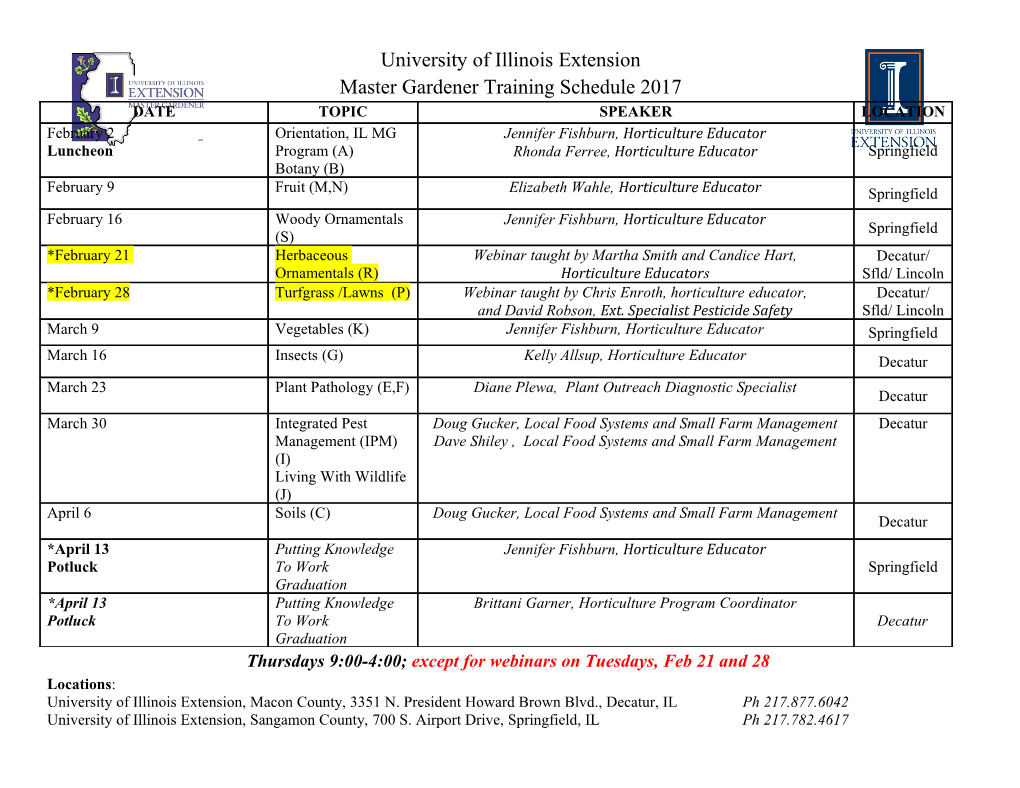
18.785 Number theory I Fall 2015 Lecture #4 09/22/2015 4 Etale´ algebras, norm and trace, Dedekind extensions 4.1 Separability We recall some standard facts about separable and inseparable field extensions and de- fine the more general notion of an ´etalealgebra (or separable algebra). This is optional background material that may be skipped by those already familiar with it. Definition 4.1. A nonzero polynomial f over a field K is separable if the zeros of f are distinct in every extension of K; equivalently, gcd(f; f 0) = 1.1 Warning 4.2. Older texts (such as Bourbaki) define a polynomial in K[x] to be separable if all of its irreducible factors are separable (under our definition); so (x − 1)2 is separable under this definition but not under ours. The older definition has the disadvantage that it is not preserved under field extension (for example, a polynomial that is inseparable as an element of K[x] becomes separable when viewed as an element of K[x], since it splits into linear factors in K[x] and every linear polynomial is separable). This discrepancy does not impact the definition of separable element or field extensions. Definition 4.3. Let L=K be an algebraic field extension. An element α 2 L is separable over K if it is the root of a separable polynomial in K[x] (in which case its minimal polynomial is separable). The extension L=K is separable if every α 2 L is separable over K; otherwise it is inseparable. Lemma 4.4. Let K be a field. If f 2 K[x] is irreducible but not separable then f 0 = 0. Proof. Let f 2 K[x] be irreducible, and let L be its splitting field over K. If f is not separable then f and f 0 have a common root α 2 L. The minimal polynomial g 2 K[x] of α over K divides f, but f is irreducible and nonzero, so deg g = deg f. But g also must divide f 0, and deg f 0 < deg f, so f 0 = 0. Corollary 4.5. Let p be the characteristic of the field K and let f 2 K[x] be irreducible. If p = 0 then f is separable, and if p > 0 then f is not separable if and only if it is of the form f = g(xp), where the polynomial g 2 K[x] is uniquely determined by f. Proof. Easy exercise. Corollary 4.6. If char K = 0 then every algebraic extension of K is separable. Corollary 4.7. Let K be a field of characteristic p ≥ 0. Every irreducible f 2 K[x] is of the form g(xpn ) for some irreducible separable polynomial g 2 K[x] and integer n ≥ 0 that are uniquely determined by f. Proof. We proceed by induction on deg f. If f is separable the corollary holds with g = f and n = 0. Otherwise p > 0 and f = g(xp) for some unique irreducible g 2 K[x] with deg g < deg f. By induction on n = deg f, we have g = h(xpn ) for some unique irreducible separable polynomial h 2 K[x] and integer n ≥ 0 and then f = h(xpn+1 ). 1Here f 0 denotes the formal derivative of f in K[x] and the gcd is defined only up to a unit. Lecture by Andrew Sutherland Lemma 4.8. Let L = K(α) be an algebraic extension contained in an algebraic closure K of K and let f 2 K[x] be the minimal polynomial of α over K. Then # HomK (L; K) = #fβ 2 K : f(β) = 0g ≤ [L : K]; with equality if and only if α is separable over K. Proof. Each element of HomK (L; K) is uniquely determined by the image of α, which must be a root β of f(x) in K. The number of these roots is equal to [L : K] = deg f precisely when f and therefore α is separable over K. Definition 4.9. Let L=K be a finite extension. The separable degree of L=K is [L : K]s := # HomK (L; K): The inseparable degree of f is [L : K]i := [L : K]=[L : K]s We will shortly show that [L : K]i is always a positive integer (in fact a power of the characteristic of K), but it follows immediately from the definition that [L : K] = [L : K]s[L : K]i: Remark 4.10. As with the degree of a field extension (defined as the dimension of a vector space), one can define the separable degree of an arbitrary algebraic extension as the cardinality of the set HomK (L; K). Multiplication of degrees is then multiplication of cardinals (if S and T are sets the product of their cardinalities is the cardinality of the set Hom(S; T ) = ff : S ! T g). Lemma 4.8 implies that for simple algebraic extensions L = K(α) we always have [L : K]s ≤ [L : K], with equality if and only if α is separable (which we will shortly show is equivalent to L=K being separable). Theorem 4.11. Let φK : K ! Ω be a homomorphism from a field K to an algebraically closed field Ω, and let L=K be algebraic. Then φK extends to a homomorphism φL : L ! Ω. Proof. We use Zorn's lemma. Define a partial ordering on the set F of pairs (F; φF ) for which F=K is a subextension of L=K and φF : F ! Ω extends φK by defining (F1; φF1 ) ≤ (F2; φF2 ) whenever F2 contains F1 and φF2 extends φF1 . Given any totally ordered subset C of F, S let E = fF :(F; φF ) 2 Cg and define φE : E ! Ω by φE(x) = φF (x) for x 2 F ⊆ E (this does not depend on the choice of F because C is totally ordered). Then (E; φE) is a maximal element of C, and by Zorn's lemma, F contains a maximal element (M; φM ). We claim that M = L. If not, then pick α 2 L−M and consider the field F = M[α] ⊆ L properly containing M, and extend φM to 'F : F ! Ω be letting φF (α) by any root of αM (f) in Ω, where f 2 M[x] is the minimal polynomial of α over M and αM (f) is the image of f in Ω[x] obtained by applying 'M to each coefficient. Then (M; φM ) is strictly dominated by (F; φF ), contradicting its maximality. 18.785 Fall 2015, Lecture #4, Page 2 Lemma 4.12. Let L=F=K be a tower of finite extensions. Then # HomK (L; K) = # HomK (F; K)# HomF (L; K): Proof. We decompose L=F=K into a tower of simple extensions and proceed by induction. The result is trival if L = K and otherwise it suffices to consider K ⊆ F ⊆ F (α) = L, where K = F in the base case. Theorem 4.11 allows us to define a bijection HomK (F; K) × HomF (F (α); K) ! HomK (F (α); K) ^ ^ ^−1 that sends (φ1; φ2) to φ: L ! K defined by φjF = φ1 and φ(α) = (φ1φ2φ1 )(α), where φ^1; φ^2 2 Aut(K) denote arbitrary extensions of φ1; φ2 to K; note that φ(α) does not depend on these choices and is a root of φ(f), where f 2 F [x] is the minimal polynomial of α and ^−1 ^^ φ(f) is its image in φ(F )[x]. The inverse bijection is φ1 = φF and φ2(α) = (φ1 φφ1)(α). Corollary 4.13. Let L=F=K be a tower of finite extensions. Then [L : K]s = [L : F ]s[F : K]s [L : K]i = [L : F ]i[F : K]i Proof. The first equality follows from the lemma and the second follows from the identities [L : K] = [L : F ][F : K] and [L : K] = [L : K]s[L : K]i. Corollary 4.14. Let L = K(α) be an algebraic extension. Then L=K is separable if and only if α is separable over K, equivalently, if and only if [L : K]s = [L : K]. Proof. If L=K is separable then α is separable over K and [L : K]s = [L : K], by Lemma 4.8. Now suppose α is separable; then [L : K]s = [L : K], by Lemma 4.8. For any β 2 L we can write L = K(β)(α), and note that α is separable over K(β), since its minimal polynomial over K(β) divides it minimal polynomial over K, which is separable. So we also have [L : K(β)]s = [L : K(β)]. The equalities [L : K] = [L : K(β)][K(β): K] [L : K]s = [L : K(β)]s[K(β): K]s then imply [K(β): K]s = [K(β): K], so β is separable over K, by Lemma 4.8. Corollary 4.15. Let L=K be a finite extension. Then [L : K]s ≤ [L : K] with equality if and only if L=K is separable. Proof. We have alredy established this for simple extensions, and otherwise we my decom- pose L=K into a finite tower of simple extensions and proceed by induction on the number of extensions, using the previous two corollaries at each step. Corollary 4.16. If L=F=K is a tower of finite extensions with L=F and F=K separable then L=K is separable. Proof. This follows from Corollaries 4.13 and 4.15.
Details
-
File Typepdf
-
Upload Time-
-
Content LanguagesEnglish
-
Upload UserAnonymous/Not logged-in
-
File Pages13 Page
-
File Size-