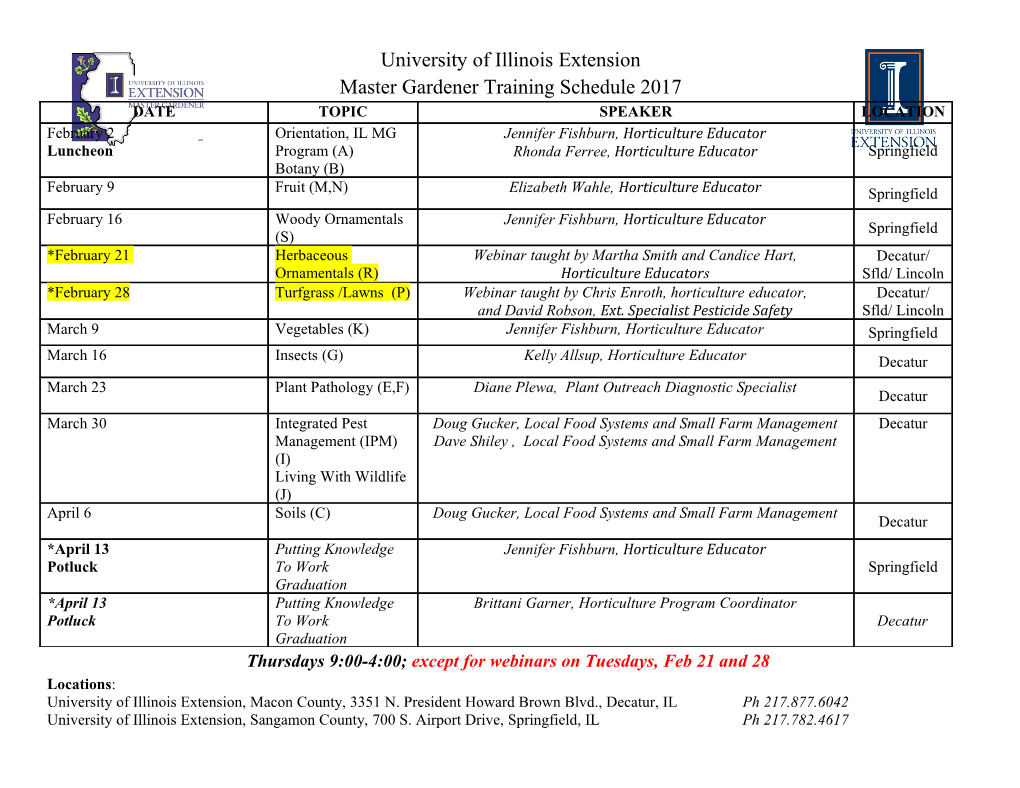
Journal of Computer-Aided Molecular Design https://doi.org/10.1007/s10822-018-0134-x PERSPECTIVE Force field development phase II: Relaxation of physics-based criteria… or inclusion of more rigorous physics into the representation of molecular energetics A. T. Hagler1,2 Received: 5 March 2018 / Accepted: 18 July 2018 © Springer Nature Switzerland AG 2018 Abstract In the previous paper, we reviewed the origins of energy based calculations, and the early science of FF development. The initial efforts spanning the period from roughly the early 1970s to the mid to late 1990s saw the development of methodolo- gies and philosophies of the derivation of FFs. The use of Cartesian coordinates, derivation of the H-bond potential, different functional forms including diagonal quadratic expressions, coupled valence FFs, functional form of combination rules, and out of plane angles, were all investigated in this period. The use of conformational energetics, vibrational frequencies, crystal structure and energetics, liquid properties, and ab initio data were all described to one degree or another in deriving and vali- dating both the FF functional forms and force constants. Here we discuss the advances made since in improving the rigor and robustness of these initial FFs. The inability of the simple quadratic diagonal FF to accurately describe biomolecular energetics over a large domain of molecular structure, and intermolecular configurations, was pointed out in numerous studies. Two main approaches have been taken to overcome this problem. The first involves the introduction of error functions, either exploit- ing torsion terms or introducing explicit 2-D error correction grids. The results and remaining challenges of these functional forms is examined. The second approach has been to improve the representation of the physics of intra and intermolecular interactions. The latter involves including descriptions of polarizability, charge flux aka geometry dependent charges, more accurate representations of spatial electron density such as multipole moments, anisotropic nonbond potentials, nonbond and polarization flux, among others. These effects, though not as extensively studied, likely hold the key to achieving the rigor- ous reproduction of structural and energetic properties long sought in biomolecular simulations, and are surveyed here. In addition, the quality of training and validation observables are evaluated. The necessity of including an ample set of energetic and crystal observables is emphasized, and the inadequacy of free energy as a criterion for FF reliability discussed. Finally, in light of the results of applications of the two approaches to FF development, we propose a “recipe” of terms describing the physics of inter and intramolecular interactions whose inclusion in FFs would significantly improve our understanding of the energetics and dynamics of biomolecular systems resulting from molecular dynamics and other energy based simulations. Keywords Force fields · Force field derivation · Potential functions · Van der Waals · Hydrogen bond · Drug discovery · Molecular dynamics · Molecular mechanics · Protein simulation · Molecular simulation · Nonbond interactions · Combination rules · Polarizability · Charge flux · Nonbond flux · Polarizability flux · Free energy · Coupling terms · Cross terms · AMBER · CHARMM · OPLS · GAFF · AMOEBA · SDFF · CFF · VFF · Consistent force field · Electrostatics · Multipole moments · Anisotropic nonbond potentials · Quantum derivative fitting · QDF Abbreviations AG Arithmetic–geometric Ala Alanine AMBER Assisted model building with energy * A. T. Hagler refinement [email protected] AMOEBA Atomic multipole optimized energetics 1 Department of Chemistry, University of Massachusetts, for biomolecular applications Amherst, MA 01003, USA AUE Absoluter unsigned error 2 Present Address: Valis Pharma, San Diego, CA, USA BCC Bond charge correction Vol.:(0123456789)1 3 Journal of Computer-Aided Molecular Design BNS Ben Naim–Stillinger OPLS-AA OPLS all atom C22 CHARMM22 OPLS-AA OPLS-AA/L OPLS all atom FF (L for CASP Critical assessment of protein structure LMP2) prediction PCILO Perturbative configuration interaction CFF Consistent force field using localized orbitals CHARMM Chemistry at HARvard Macromolecu- PDB Protein data base lar Mechanics PEFC Potential Energy Function Consortium CHELPG Charges from electrostatic potentials (Biosym) using a grid-based method PMF Potential of mean force CHEQ Charge equilibration method POL3 Polarizable water model (3) CMAP Grid based energy correction map PPII Polyproline II conformation CNDO Complete neglect of differential QCPE Quantum chemistry program exchange overlap QDF Quantum derivative fitting COMPASS Condensed-phase optimized molecu- QDP Charge dependent polarizability lar potentials for atomistic simulation QM Quantum mechanics studies RESP Restrained electrostatic potential CVFF Consistent valence force field RMS Root mean square DFT Density functional theory RMSD Root mean square deviation DMA Distributed multipole analysis RMSE Root mean square error DZVP Valence double-zeta plus SAMPL Statistical assessment of the modeling polarization of proteins and ligands (competition) ECEPP Empirical conformational energy pro- SAXS Small angle X-ray scattering gram for peptides SCF-LCAO-MO Self-consistent field-linear combina- EHT Extended Hückel theory tion of atomic–molecular orbital ESP Electrostatic potential SDFF Spectroscopically determined force EVB Empirical valence bond fields (for macromolecules) FEP Free energy perturbation SIBFA Sum of interactions between fragments FF Force field ab initio (computed) FQ Fluctuating charges SPC Simple point charge (water model) FRET Forster resonance energy transfer ST2 Four point water model replacing Ben- GAFF General AMBER force field Naim Stillinger (BNS) model Gly Glycine STO Slater-type atomic orbitals GROMOS GROningen MOlecular Simulation SWM4-NDP Simple water model with negative hCatL human Cathepsin L Drude polarization HF-SCF Hartree–Fock self-consistent-field TIP3P Transferable intermolecular potential Hyp Hydroxyproline three point IDP Intrinsically disordered protein TTBM Tri-tert-butylmethane LCAO Linear combination of atomic orbitals TZVP Triple-zeta plus valence polarization LJ Lennard-Jones (basis set) LP Oxygen lone pair UB Urey–Bradley MC Monte Carlo UBFF Urey Bradley force field MCMS FF Momany, Carruthers, McGuire, and VDW Van der Waals Scheraga force field VFF Valence force field MCY Matsuoka–Clementi–Yoshimine WH Waldman–Hagler MD Molecular dynamics MDDR MDL drug data report 1 Introduction MDL Molecular Design Limited MEP Molecular electrostatic potentials 1.1 Rigorous optimization of the 12-6-1 quadratic MM Molecular mechanics diagonal FF, and characterization of its capabili- MMFF Merck molecular force field ties and deficiencies NMA N-methylacetamide 1.2 Simple 12-6-1 quadratic diagonal FFs (as used in OPLS Optimized potential for liquid standard biomolecular FFs) are not adequate to simulations achieve quantitative accuracy 1 3 Journal of Computer-Aided Molecular Design 1.3 Abandoning representations based on Physics for 3.7 Thermodynamic data is essential in assessing the hybrid physics-based/empirical FFs. Polarizability validity of FFs and Empirical correction factors 3.7.1 Summary: physics in current 12‑6‑1 fixed charge FFs is incapable of reliably 2 AMBER—empirical adjustments: on tweaking of accounting for peptide and protein proper‑ torsional parameters to compensate for deficiencies ties in representation of physics 3.8 Drude polarizable FF 2.1 ff14ipq/ff15ipq 3.8.1 A major concern—omission of flexible 2.1.1 J‑coupling and secondary structure pro‑ geometry and charge flux pensities—convergence issues 3.8.2 Hydrocarbons 2.1.2 Protein stability—trajectories longer than 3.8.3 Observation of consequences of omission 10 μs required of charge flux 3.8.4 Internal parameters 2.2 AMBER-FB15/TIP3P-FB 3.8.5 Benzene 3.8.6 Extension of Drude FF to additional func‑ 2.2.1 Stability of seven proteins over short trajec‑ tional groups and proteins tories 3.8.7 Danger of deriving a FF from one or two 2.2.2 Temperature dependence of secondary compounds structure in two peptides 3.8.8 A reparametrization of the amide FF 2.2.3 CMAP in AMBER 3.9 Drude-2013 protein force field 2.3 Energetics, crystal structures and sublimation ener- gies: a forgotten and missed powerful resource 3.9.1 A third reparametrization of the amide FF 2.4 AMBER—introducing polarizability 3.9.2 Polarizability results in degradation of Fit 2.5 On the use of different data sets: a problem that permeates the FF development field and sabotages 3.10 Summary of Drude FF development for organic rigorous comparisons of the accuracy of FFs compounds and proteins. Polarizability does not lead to a general improvement of FF perfor- 3 CHARMM—empirical adjustments: introduction of mance—often degrades fit grid-based correction factors 3.10.1 Inadequate training sets can lead to future 3.1 CHARMM22/CMAP failures and need for reparametrization 3.2 Rigorously comparing FFs 3.11 CHARMM polarizable charge equilibration FF 3.2.1 Lysozyme 3.3 C22/CMAP—extension to other families 3.11.1 Significant deviations remain, indicating 3.4 On the immense value and validityof experimen- flaws in CHEQ FF tal crystal structure and thermodynamics for FF 3.11.2 CHEQ and other FFs (other than OPLS) assessment have difficulty accounting for energetics 3.5 C36: further reparametrization of sidechain torsion of tetra‑ala conformers potentials and the CMAP correction
Details
-
File Typepdf
-
Upload Time-
-
Content LanguagesEnglish
-
Upload UserAnonymous/Not logged-in
-
File Pages60 Page
-
File Size-