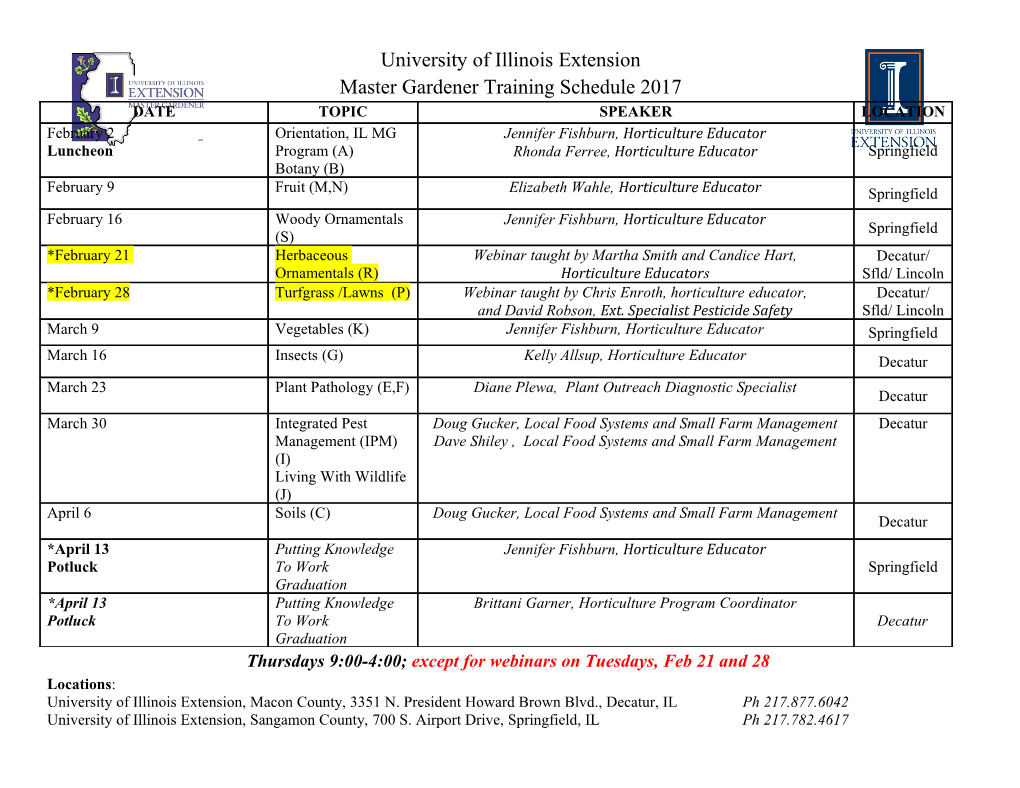
Perspectives on Modeling the Transport of Water and its Distribution at the Lunar Poles Parvathy Prem, Dana M. Hurley & G. Wesley Patterson August 9th, 2018 Lunar Polar Volatiles Background: Image of the Moon [Credit: NASA] superimposed on a sequence of New Horizons images of Jupiter’s volcanically active moon, Io [Credit: NASA/JHUAPL/SwRI] Introduction • Remote sensing observations have been accompanied by the development of numerical models of volatile transport/sequestration. Crider & Vondrak, 2000 Left to right: LROC South Polar mosaic [NASA/GSFC/ASU]; Diviner brightness temperature [Paige et al., 2010]; LEND neutron suppression [Sanin et al., 2017; different spatial scale]; LAMP off/on-band albedo ratio [Hayne et al., 2015]. • Themes in this presentation: - What role can numerical models play in addressing outstanding questions regarding the origin, nature and distribution of polar volatiles? - What assumptions and uncertainties underlie model results? How can these be addressed? Lunar Polar Volatiles, August 7-9, 2018 [email protected] 1 / 17 Outline • Contemporary, continuous sources of volatiles; • Ancient, episodic sources of volatiles; • What is the distribution of polar volatiles? (focusing mostly on water, as a volatile of interest) Background: ‘Portrait of a Crater’, Alan Bean Lunar Polar Volatiles, August 7-9, 2018 [email protected] 2 / 17 How Would A Water Vapor Exosphere Behave? • Several potential source mechanisms: solar wind [e.g. Schorghofer et al., 2017], micrometeoroid impacts [e.g. Hurley & Benna, 2017], remobilization of polar water [e.g. Farrell et al., 2013]. • Characteristic features: - Dawn-dusk asymmetry; - Higher exospheric column density, larger (absolute) diurnal variations at higher latitudes [Prem et al., 2018; Schorghofer et al., 2017]. H2O above surface H2O on surface [adapted from Schorghofer et al., 2017] Dusk Lunar Polar Volatiles, August 7-9, 2018 [email protected] 3 / 17 Modeling Molecular Mobility • Different approaches – all 1 monolayer/s similarly sensitive to surface temperature. 1 monolayer/lunar day • Distribution of activation energies [Farrell et al., 2016]. 1 monolayer/109 years • Distribution of small-scale surface temperatures [Bandfield et al., 2015; Prem Andreas, 2007 푑푁 푝푣푎푝 = Schorghofer, 2014 et al., 2018]. 푑푡 2휋푚푘퐵푇 Sandford et al., 1993 • Coverage-dependence 푑푁 −퐸푎 Ea = 0.5 eV* = ν ∙ exp 푁 [Farrell et al., 2016]. 푑푡 푘퐵푇 Ea = 0.7 eV* *Farrell et al., 2016 (LPSC) • Implications for volatile transport due to different source mechanisms and on different bodies. Lunar Polar Volatiles, August 7-9, 2018 [email protected] 4 / 17 Diurnal and Spatial Variations • Orbital-scale to rover-scale variations. • Diurnal cycling of cold trapping rates [Prem et al., 2018]. • Influence of topography [e.g. Schorghofer et al., 2017, Hodges & Mahaffy, 2016]. • Can collisionless migration lead to non-uniform cold-trapping? Moores (2016) finds preferential deposition at lower latitude cold traps; other analyses differ. Lunar Polar Volatiles, August 7-9, 2018 [email protected] 5 / 17 The Once and Future Lunar Exosphere • Almost any landed lunar mission will be an active volatile release experiment. - Models and observations are critical to constraining the degree of contamination and to understanding how volatiles interact with the lunar regolith. • Parameters that govern gas-surface interactions: - Sticking coefficient; - Desorption activation energy; - Degree of thermalization. Critical to understanding transport to the poles. sticking coefficient = 1 = coefficient sticking • How do model parameters affect t = 0 nominal spacecraft trajectory spacecraft observables? t = 1 s sticking coefficient = 0 = coefficient sticking Lunar Polar Volatiles, August 7-9, 2018 [email protected] 6 / 17 Outline • Contemporary, continuous sources of volatiles; • Ancient, episodic sources of volatiles; • What is the distribution of polar volatiles? (focusing mostly on water, as a volatile of interest) Background: ‘Portrait of a Crater’, Alan Bean Lunar Polar Volatiles, August 7-9, 2018 [email protected] 7 / 17 Ghosts of Atmospheres Past • How do we read the geological record written at the lunar poles? Are there tell- tale traces of volcanically- or impact-generated atmospheres? • Volatile transport, loss and deposition mechanisms may be fundamentally different in transient, collisional atmospheres (transition at 106 kg). - Gas dynamic pressure (winds, hydrodynamic escape). - Opacity to thermal, ultraviolet radiation. - Atmospheric structure (e.g. shocks). - Gas-phase and surface chemistry. • Why investigate comets? - Water (if present) appears to be non-uniformly distributed among cold traps [e.g. Mitrofanov et al., 2010, Gladstone et al., 2012] – difficult to achieve this through continuous source mechanisms. - Detection by LCROSS of CH4, NH3 and other compounds (besides H2O) at Cabeus crater [Colaprete et al., 2010]. - Isolated sub-surface hydrogen signatures at some cold traps [Miller et al., 2014]. - Why do the poles of Mercury look so different? [Lawrence, 2017] Lunar Polar Volatiles, August 7-9, 2018 [email protected] 8 / 17 Post-Impact Volatile Transport • Highlights [Prem et al., 2015 and Prem et al. (under review)]: - Characteristic structure – antipodal, day-side shocks, pressure-driven low-altitude winds. Night Day - Self-shielding from photodestruction is important. - Atmosphere lifetime of several lunar days (10 days for initial mass 1011 kg; Stewart, 2010). - Volatiles may be non-uniformly distributed between poles and between cold traps. - Impact parameters are important. - Dense regions of the atmosphere tend to also be warm. • Key outstanding questions: - Gas-dynamic and chemical interactions between multiple species – does the composition of cold-trap deposits mirror that of the impactor? - Long-term evolution and dependence on impact parameters. - Comets vs. hydrated asteroids [e.g. Svetsov & Shuvalov, 2015]. Lunar Polar Volatiles, August 7-9, 2018 [email protected] 9 / 17 Volcanically-Generated Atmospheres • Different compositional signatures and distribution? Mostly CO (Tv ~18 K) and S (Tv ~200 K) [Tv from Zhang & Paige, 2009]. • To make an atmosphere from scratch… at 0.1 Ga intervals [from Wilson & Head, 2018] [from Needham & Kring, 2017] Peak lunar magma volume flux, 106 m3/s → 3 x 105 kg/s volatile release rate (for magma density 3 g/cm3; 100 ppm volatile content). Lunar Polar Volatiles, August 7-9, 2018 [email protected] 10 / 17 Volcanically-Generated Atmospheres Background: Galileo image of Io, 1997 [Galileo Project/JPL/NASA] Peak lunar magma volume flux, 106 m3/s → 3 x 105 kg/s volatile release rate (for magma density 3 g/cm3; 100 ppm volatile content). For perspective, Pele/Pillan on Io are estimated 6 to release ~10 kg/s SO2 [Cataldo et al., 2002]. Walker et al., 2010 – numerical modeling. Lunar Polar Volatiles, August 7-9, 2018 [email protected] 11 / 17 Outline • Contemporary, continuous sources of volatiles; • Ancient, episodic sources of volatiles; • What is the distribution of polar volatiles? (focusing mostly on water, as a volatile of interest) Background: ‘Portrait of a Crater’, Alan Bean Lunar Polar Volatiles, August 7-9, 2018 [email protected] 12 / 17 Testing Models of Volatile Transport/Deposition… • …is complicated by the fact that the distribution and physical form of water at the lunar poles remain to be definitively characterized. • Determining depth-distribution is critical. • Bistatic radar measurements offer unique mapping capabilities. - Multiple levers; incidence & bistatic angles, wavelength. LRO [Patterson et al., 2017] Earth-based radar • Complementary to other surface/sub-surface measurement techniques. Ratio Polarization Circular Bistatic angle (°) Lunar Polar Volatiles, August 7-9, 2018 [email protected] 13 / 17 The Curious Case of Cabeus Crater • Mini-RF bistatic observations show an opposition surge over the floor of Cabeus, but… • …the radar response at Cabeus appears to depend on both phase (bistatic) and incidence angle. • What does this tell us about the abundance & distribution of water? (How do incidence angle and regolith properties affect the nature of the opposition effect?) • The plot thickens [Patterson et al., 2018]… - Opposition response is not apparent at X- band wavelengths (4.2 cm). - Qualitatively different opposition response Top: Mini-RF/Arecibo bistatic data Bottom: Mini-RF monostatic (β=0°) data is seen for the illuminated floor of Amundsen in the X-band. Lunar Polar Volatiles, August 7-9, 2018 [email protected] 14 / 17 Monte Carlo Radiative Transfer in Ice/Regolith • Monte Carlo models of radiative transport have broad applicability [e.g. Lawrence et al., 2006 (LPNS), de Wet et al., 2018 (CRaTER)]. • Modeling the Coherent Backscatter Opposition Effect at radar wavelengths: constructive interference between time-reversed paths → coherent backscatter. Lunar Polar Volatiles, August 7-9, 2018 [email protected] 15 / 17 Monte Carlo Radiative Transfer in Ice/Regolith • Monte Carlo models of radiative transport have broad applicability [e.g. Lawrence et al., 2006 (LPNS), de Wet et al., 2018 (CRaTER)]. • Modeling the Coherent Backscatter Opposition Effect at radar wavelengths: ice regolith 2λ ice over regolith under 0.5λ regolith 4λ ice ″ • Opposition response is highly sensitive to structure of the shallow subsurface – more so at oblique incidence. Lunar Polar Volatiles, August 7-9, 2018 [email protected] 16 / 17 Where Do We Go From Here? • What is the abundance, distribution and physical form of water and other volatiles at the lunar poles? How do we synthesize existing datasets, and what new observations (and models) are needed? • How do we read the geological record written at the lunar poles? What processes have written and rewritten this record over time? • Why are the poles of the Moon and Mercury so different? Background: Simulated polar lighting conditions at the NASA Ames Lunar Lab testbed [Credit: NASA; Wong et al., 2017] Lunar Polar Volatiles, August 7-9, 2018 [email protected] 17 / 17.
Details
-
File Typepdf
-
Upload Time-
-
Content LanguagesEnglish
-
Upload UserAnonymous/Not logged-in
-
File Pages18 Page
-
File Size-