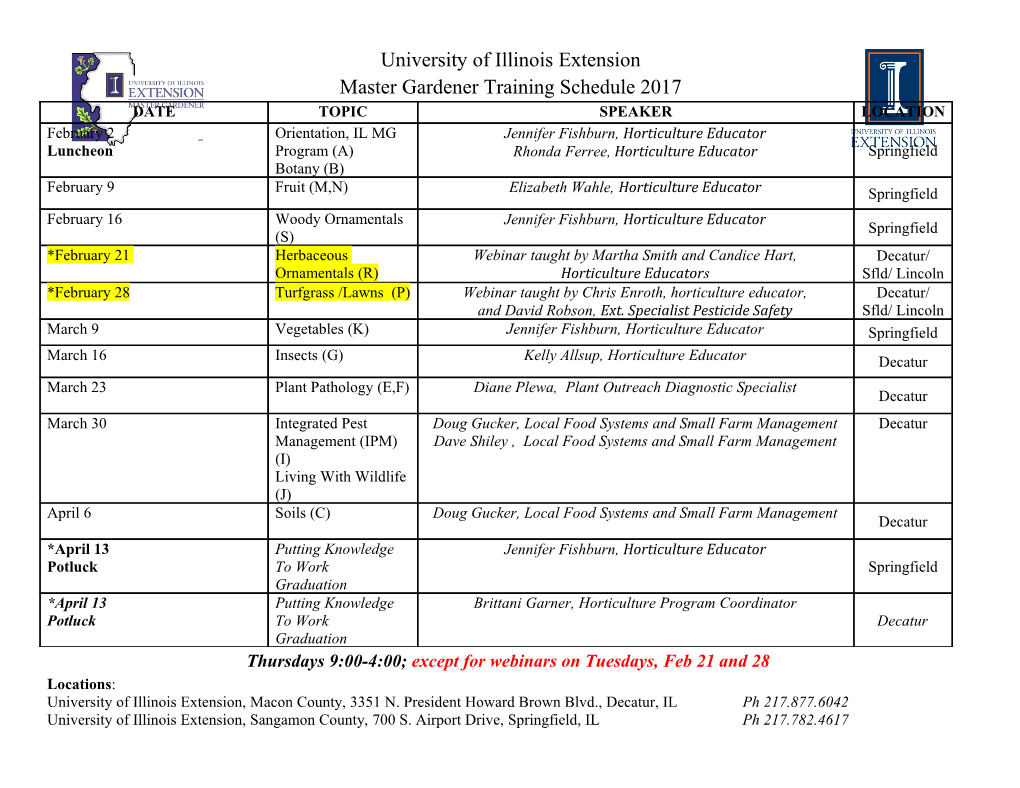
STABLE AND SEMI-STABLE PARTICLES The Positron: Particles and Antiparticles Until 1932 the only elementary particles known in physics were the proton, the electron and the photon which we called stable particles. In that year two new elementary particles were discovered, viz. the neutron and the positron. The existence of a positron had been predicted by Dirac in 1930 in his relativistic theory of the free electron. Dirac set up the relativistic wave equation for the electron and showed that solutions were possible for all values of the total energy Et whenever where m is the actual mass of the moving electron and c is the velocity of light. According to the Dirac theory there exists a set of mathematically possible positive energy states with energies greater than mc2 and also a set of possible negative energy states with energies less than - mc2. the following simple argument may help the reader. The relativistic equation connecting the momentum and total energy of a moving particle is where mo is the rest mass of the moving particle and p is its momentum. Thus and for any given value of the momentum p the energy Et can be positive or negative. In the case of the electron there are therefore positive energy states corresponding to observable energies, but the negative energy states have no simple physical meaning and can only be interpreted mathematically. Since electrons in the positive states would make radiative transitions to the negative states, and since this is not observed, Dirac proposed that all the negative energy states in a perfect vacuum were completely occupied by electrons, whereas all the positive states were normally empty. The negative states are therefore completely filled and are unobservable until a vacancy occurs in one of them by the removal of an electron to a positive, observable, energy state by the interaction of the electron with the electromagnetic field. This leaves a positively charged hole or vacancy which is manifest as a particle with the same mass as its companion electron but with opposite charge and spin (conservation 9 of angular momentum). The energy required for this upward transition will be, since two particles are created, which means that a positron-electron pair cannot be created by bombarding particles or photons of less than the threshold energy of 1·02 MeV, see Fig. 26.5. Negative·· electron O observable Energy E / E•mc2 i I AE•2 mc2 )0 }E=-mc2 0 0 0 0 0 0 0 0 0 Electrons 0 0 0 0 0 0 (. ) 'J 0 0 0 of negative mass 0 0 0 0 0 0 l.J .) � 0 0 unobservable 0 0 0 0 (_) 0 0 (.,21 0 0 0 l J to Continuous -: distribution + Vacancy a positron extends to Infinity Fig. 26.5 Creation of a positron-electron pair. according to Dirac. The above is a simplified description of the formation of a positron-electron pair. In free flight most positrons are slowed down to low energies before annihilation and many of these are then captured by electrons to give so-called positronium atoms. A positronium atom is a short-lived positron-electron pair. The relativistic equation for E, holds for all free particles of spin fh/21t, so that all such particles have 'antiparticles'. When a.particle and its antiparticle meet, great energy is created by their mutual annihilation. As we have seen, the positron-electron pair requires about 1 Me V for its creation so that we can expect the creation of a proton-antiproton pair to require at least 1836 x 1 MeV, i.e. an energy of nearly 2 Ge V relative to the centre of the mass of the pair. Calculation shows that the initial kinetic energy of the bombarding proton in a proton• proton collision producing an antiproton by p" +p+ -+(p+ +p+)+(p+ +p+) is 6mPc2 = 5·6 GeV, when allowance is made for conservation laws and relativistic effects at these high energies. 10 Particles and antiparticles have opposite charges but always have the same mass. They interact strongly in pairs where they create great energy from the annihilation of particle matter. The existence of the antiproton and the antineutron as well as the positron gives strong support to the Dirac theory of free particles. Fig. 26.7 Emulsion photograph showing annihilation of anti proton. The antiproton enters from the top left-hand corner and is annihilated at the end of its range. The annihilation energy is then distributed among the secondary charged particles of which four pions and two protons are shown. (From Powell, ibid.) 11 Pions, Muons and Kaons We have mentioned that primary cosmic rays consist largely of protons. When such fast protons encounter the nuclei of atoms in the atmosphere, high-energy nucleon-nucleon collisions take place which cannot easily be reproduced in the laboratory. It is not surprising, then, that many new particles were discovered in cosmic ray events, particles with very strange properties compared with the early elementary particles known to physicists (stable particles). As we have said the first new particle to be found was the positron, soon to be followed by the -meson, which has a mass lying between that of the electron and the proton. Many other mesons have since been discovered, and now we know that when a fast cosmic ray proton strikes a nucleus it reacts strongly with the nucleons and in the ensuing rearrangement a shower of many mesons can be ejected. The discovery of the first meson as an elementary particle was made in 1937 by Neddermeyer, Anderson, Street, and Stevenson in Wilson cloud chamber cosmic ray observations. This particle was then called a mesotron and could be either positive or negative. We now call this particle the -meson or muon. Its mass was estimated to be about three hundred times the mass of the electron. The existence of a particle with these peculiar characteristics had been predicted by Yukawa in 1935. Yukawa put a theory of nuclear attraction forces which required the existence of a particle, with either positive or negative charge, and of mass equivalent to two or three hundred electronic masses, to give the correct distance over which the short range forces act, viz. about 1 fm. These mesons of Yukawa are the quanta of the nuclear force-field and it was natural to identify the 1937 experimental meson with Yukawa's nuclear photon. This was then interpreted as the strong force-carrier within the nucleus, shuttling backward and forwards among the nucleons and so binding them together. One of the properties of the negative meson, as predicted by the theory, was that when stopped by ordinary solid materials it should be absorbed very rapidly, showing strong nuclear interaction, but in time it was found that -mesons have only a weak interaction with nuclei, a result incompatible with Yukawa's requirements. Experimentally it was found that fast -mesons could pass through thick plates of lead without being absorbed, showing their weak interactions with nuclei of solid substances. Hence the possibility of identifying the experimental • meson with Yukawa's particle was open to doubt, and when in 1947 the first nuclear emulsion plates exposed to cosmic rays at high altitudes were examined by C. F. Powell and his team at Bristol, and the existence of another meson of mass about 300 me was suspected, this doubt was strengthened, since the Yukawa theory did not postulate two different mesons. In the early nuclear emulsion plates some of the mesons were 12 found to decay at the end of their range. These were later identified as positive -mesons which decay when stopped in the emulsion to secondary mesons. The new -mesons were also shown to be produced in disintegration processes and it was found that they reacted very strongly with the nucleons of the emulsion nuclei. I I I I I _..,._ Fig. 26.2 First observation of a-meson decay in a nuclear emulsion. The pion enters the plate at the bottom left-hand corner and reaches the end of its range at the top. A secondary µ-meson is ejected nearly backwards along the line of approach of the pion. Note the increase of grain density of these particles at the end of their respective ranges: (From PowcH, ibid.) We have mentioned that -mesons (pions), which have mass about 273 me, are created when primary cosmic ray protons collide with atmospheric nuclei and cause energetic nuclear disintegrations. Pions are radioactive and have a very short lifetime T: They can exist in all three states: n ", with T= 25 ns 1t , with T-25 ns n°, with T'=O·l fs. 13 These lifetimes, which refer only to mesons at rest, are so short, that only a fraction of cosmic ray pions can reach sea-level. They are attenuated in the atmosphere because of the strong nuclear interaction. The charged pions decay to muons and neutrinos as follows: n+ --.µ+ +v+33 MeV n---.µ-+v+33 MeV. The charged muons are also unstable, emitting electrons: µ±--.e± +2v+ 105 MeV. The radioactive decay of -mesons can be followed easily in the nuclear emulsion plate. The above equations are of great importance. Since the muon decays from rest, the conservation of linear momentum requires that two particles must be ejected. The only particle observed is the electron with its continuous energy spectrum. Since the charge is conserved, one expects the second particle to be a neutrino. However, since the spin of the muon is ½ and the sum of the electron and neutrino spins can only be 0 or 1, but never! The conservation of angular momentum requires another particle of opposite spin 1/2 to be ejected at the same time.
Details
-
File Typepdf
-
Upload Time-
-
Content LanguagesEnglish
-
Upload UserAnonymous/Not logged-in
-
File Pages22 Page
-
File Size-