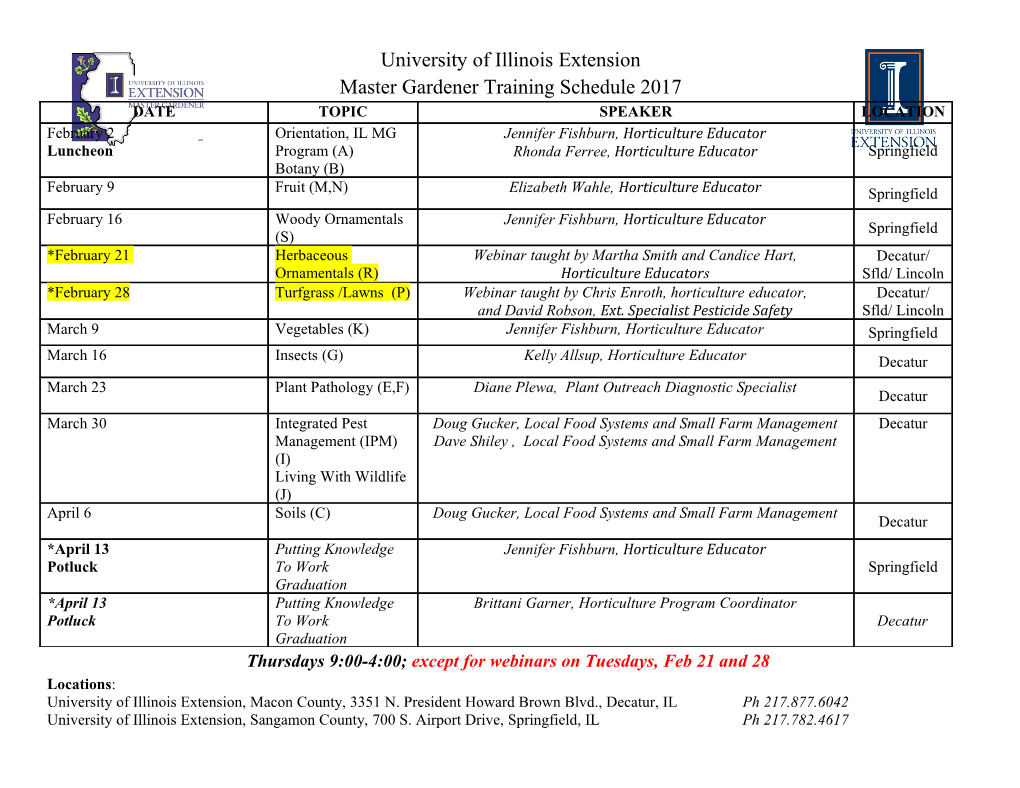
Feasibility of Spectro-Polarimetric Detection of Atmospheric Components of Exoplanets Jun Takahashi (University of Hyogo) Taro Matsuo (Osaka University) Polarimetry for planets • Reflection makes polarization. Polarization of the reflected light from a planet has information of its atmosphere, clouds and surface. • Polarimetry has been a powerful method to investigate Solar-System objects. For example, polarimetry for Venus (e.g., Lyot 1929) played a key role to identify H2SO4 hazes by determining the size and refractive index of the reflecting particles. • As is for Solar-System planets, polarimetry for exoplanets may be a good diagnostic tool to know … • atmospheric composition • cloud/haze altitude (Stam 2004, 2008) • optical thickness of atmosphere ? (Takahashi+ 2013) • existence of a surface ocean ?? (McCullough 2006) Pol. spectra of planets • Model calculations 668 D. M. Stam et al.: Polarimetry to detect and characterize Jupiter-like extrasolar planets Earth-like planet, Stam(2008) Jupiter-like planet, Stam(2004) ) 0.20 1.0 % P ( a. b. CH3 features 0.8 0.15 F 0.6 H2O clear 0.10 O2 Flux model 1 0.4 0.05 model 2 low cloud model 3 0.2 Degree of polarization Deg. of polarization, P ofpolarization, Deg. high haze 0.00 0.0 0.4 0.5 0.6 0.7 0.8 0.9 1.0 0.4 0.5 0.6 0.7 0.8 0.9 1.0 WavelengthWavelength(nm) (µm) Wavelength (µm) Fig. 4. The flux F• andThe degree enhanced of polarization featuresP of are starlight explained reflected by by the three decrease Jupiter-like in EGPs intensity for α = of90 ◦the.Planetarymodelatmosphere1 (solid lines) containsmultiply only molecules, scattered model component 2 (dashed lines) at is the simila absorptionrtomodel1,exceptforatroposphericcloudlayer,andmodel3(dotted wavelengths as compared lines) is similar to modelwith 2, that except at for the a stratospheric continuum haze wavelengths layer. • Pol spectra are sensitive to the clouds/haze altitude. and the sensitivity of the degree of polarization of the reflected For model 2, which is the atmosphere with the tropospheric stellar radiation to the structure and composition of the plane- cloud, both F and P (Fig. 4a and 4b) at the shortest wave- tary model atmosphere. lengths are similar to those of model 1, because at these wave- lengths, the molecular scattering optical thickness of the atmo- spheric layers above the cloud is so large that hardly any stellar 4. Results light will reach the cloud layer. In the strong CH4-absorption band around 0.89 µm, With increasing wavelength, the molec- 4.1. Flux and polarization as functions of wavelength ular scattering optical thickness decreases, and, at least at con- Figure 4 shows spectra of the reflected flux F and degree of po- tinuum wavelengths, the contribution of light scattered by the larization P for the 3 model atmospheres and a planetary phase cloud particles to the reflected F and P increases. The slope of the continuum F is less steep for model 2 than for model 1, angle α of 90◦. These spectra have been calculated at the same because while the molecular scattering optical thickness de- 1-nm intervals at which the CH4 absorption cross-sections have been given (Karkoschka 1994). In order to present general re- creases with wavelength, the cloud’s (scattering) optical thick- 2 2 2 2 ness increases. The decrease of the continuum P for model 2 sults in Fig. 4, we have set πB0r R /(d D )(seeEq.(5))equal 1 (Fig. 4b) is due to the increased multiple scattering within the to one. In fact, Fig. 4a thus shows 4 a1(λ, 90◦), with a1 the (1,1)-element of the planetary scattering matrix S (cf. Eq. (6)) cloud layers, as well as to the low degree of polarization of light and Fig. 4b, b (λ, 90 )/a (λ, 90 ). Using Eq. (5), scaling the that is scattered by cloud particles (see Fig. 3b). − 1 ◦ 1 ◦ fluxes presented in Fig. 4a to obtain results for a Jupiter-like In the CH4-absorption bands, F is generally larger and P extrasolar planet in an arbitrary planetary system is a straight- smaller for model 2 than for model 1, just like at continuum forward excercise. Note that P (Fig. 4b) is independent of the wavelengths. In the strong absorption band around 0.89 µm, choice of r, R, d, D,andB0,becauseP is a relative measure. however, F and P of the two models are similar, because at The flux and polarization spectra in Fig. 4 can be thought these wavelengths, hardly any stellar light can reach the cloud of to consist of a continuum with superimposed high-spectral layer due to the large molecular absorption optical thickness resolution features that are due to absorption by CH4.Recent of the atmosphere above the cloud. The light that is reflected Earth-based spectropolarimetric measurements of the gaseous at these wavelengths, has thus been scattered in the highest at- planets of our own Solar System using ZIMPOL show a similar mospheric layers, which are identical in model atmospheres 1 spectral structure (Joos et al. 2004). and 2. For model 1, which is the clear atmosphere, the continuum For model 3, the atmosphere with the tropospheric cloud F decreases steadily with λ (Fig. 4a), following the decrease and the stratospheric haze, F is at all wavelengths somewhat of the molecular scattering optical thickness with λ.Thecon- larger than for model 2, even at the shortest wavelengths, where tinuum P (Fig. 4b) increases with λ, because the smaller the model 2 is almost indistinguishable from model 1 (Fig. 4a). molecular optical thickness, the less multiple scattering takes The influence of the optically thin haze on F is explained by place within the atmosphere; and multiple scattering tends to the relatively small molecular scattering and absorption optical lower the degree of polarization of the reflected light. Multiple thickness above the high-altitude haze layer: at all wavelengths, scattering also decreases with increasing absorption by CH4. a significant fraction of the incoming stellar light reaches the For model atmosphere 1, this fully explains the high values haze layer and is reflected back to space. The degree of polar- of P within the CH4 absorption bands (Stam et al. 1999). In ization P (Fig. 4b) for model 3 is at all wavelengths signifi- the strong absorption band around 0.89 µm, P = 0.95, and thus cantly lower than that for model 1 and 2 mainly because light almost reaches its single scattering value at a single scattering that is singly scattered by the haze particles has a very low de- angle of 90◦ (which corresponds with a planetary phase angle α gree of polarization (see Fig. 3b). In particular, P is very low of 90◦), namely 0.96 (see Fig. 3b). in the strong absorption band around 0.89 µm. Whereas in the Pol. spectra of planets • Earthshine observations (Sterzik+ 2012, Miles-Paez+ 2014). A&A 562, L5 (2014) (Miles-Paez+ 2014) 18 values of the surrounding continuum, i.e., similar in intensity This work 16 Sterzik et al. 2012 to the blue optical wavelengths. Even more important should 14 Bazzon et al. 2013: highlands be the linear polarization signal of water bands at 1.4 µm Bazzon et al. 2013: maria H O ∼ 12 2 and 1.9 µm, which unfortunately cannot be characterized from ∼ 10 the ground due to strong telluric absorption. Spectropolarimetric (%) * O H O O 8 2 2 2 models of the Earth, guided by the visible and NIR observations p H O 2 H O 6 2 shown here, could provide hints to their expected polarization 4 values. To this point, we presented earthshine p values as measured 2 ∗ from the light deflection on the Moon surface. However, this 0 0.3 0.6 0.9 1.2 1.5 1.8 2.1 process introduces significant depolarization due to the back- Wavelength (µm) scattering from the lunar soil (Dollfus 1957); the true linear Enhanced features atFig. molecular 3. Our visible (H2O, and O2 NIR) absorption spectropolarimetric wavelengths measurements were of the polarization intensity of the planet Earth is actually higher. At confirmed by the observations.earthshine compared to literature data. A 10-pixel binning was applied optical wavelengths, the depolarization is estimated at a fac- ➡Spectro-polarimetryto the seems NIR spectrum to be a ofpromising region B. Themethod uncertainty to detect per wavelength is plot- tor of 3.3λ/550 (λ in nm) by Dollfus (1957),makingtruepo- atmospheric molecules.ted as vertical gray error bars. Wavelengths of strong telluric absorption larization fall in the range 26 31%. We are not aware of any have been removed. Some molecular species seen in “emission” (in- determination of the depolarization− factor for the NIR in the dicative of strong atmospheric flux absorption and less multiscattering literature. The extrapolation of Eq. (9) by Bazzon et al. (2013) processes occurring at those particular wavelengths) are labeled. The toward the NIR yields corrections of 2.2 3.3forthewave- vertical dashed line separates the ALFOSC and LIRIS data. length interval 0.9 2.3 µm, implying∼× that the−× true linear polar- ization intensity of− the Earth may be 9 12% for the NIR con- ∼ − We compare our measurements with data from the literature tinuum, and 12 36% at the peak of the O2 (1.25 µm) and ∼ − in Fig. 3. To improve the quality of the NIR linear polarization H2O(1.12µm) bands. The extrapolation of Dollfus (1957) depo- degree spectrum of region B, we applied a ten-pixel binning in larization wavelength dependency toward the NIR would yield the spectral dimension. Overlaid in Fig. 3 are the optical p val- even higher true polarization values by a factor of 4.
Details
-
File Typepdf
-
Upload Time-
-
Content LanguagesEnglish
-
Upload UserAnonymous/Not logged-in
-
File Pages19 Page
-
File Size-