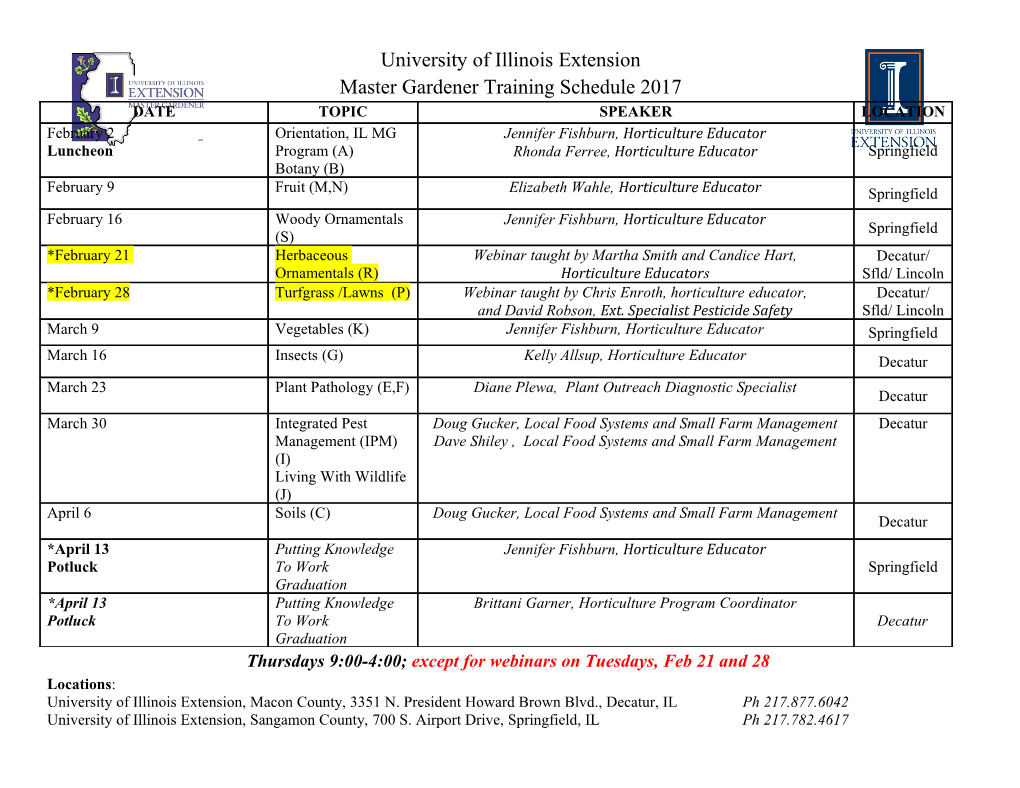
molecules Review Synthesis of DNA Origami Scaffolds: Current and Emerging Strategies 1, 1, 1, 1 1 Joshua Bush y , Shrishti Singh y , Merlyn Vargas y , Esra Oktay , Chih-Hsiang Hu and Remi Veneziano 1,2,* 1 Volgenau School of Engineering, Department of Bioengineering, George Mason University, Fairfax, VA 22030, USA; [email protected] (J.B.); [email protected] (S.S.); [email protected] (M.V.); [email protected] (E.O.); [email protected] (C.-H.H.) 2 Institute for Advanced Biomedical Research, George Mason University, Manassas, VA 20110, USA * Correspondence: [email protected] These authors contributed equally to this work. y Academic Editor: Kirill A. Afonin Received: 1 July 2020; Accepted: 24 July 2020; Published: 26 July 2020 Abstract: DNA origami nanocarriers have emerged as a promising tool for many biomedical applications, such as biosensing, targeted drug delivery, and cancer immunotherapy. These highly programmable nanoarchitectures are assembled into any shape or size with nanoscale precision by folding a single-stranded DNA scaffold with short complementary oligonucleotides. The standard scaffold strand used to fold DNA origami nanocarriers is usually the M13mp18 bacteriophage’s circular single-stranded DNA genome with limited design flexibility in terms of the sequence and size of the final objects. However, with the recent progress in automated DNA origami design—allowing for increasing structural complexity—and the growing number of applications, the need for scalable methods to produce custom scaffolds has become crucial to overcome the limitations of traditional methods for scaffold production. Improved scaffold synthesis strategies will help to broaden the use of DNA origami for more biomedical applications. To this end, several techniques have been developed in recent years for the scalable synthesis of single stranded DNA scaffolds with custom lengths and sequences. This review focuses on these methods and the progress that has been made to address the challenges confronting custom scaffold production for large-scale DNA origami assembly. Keywords: single-stranded DNA; DNA scaffolds; DNA origami; nucleic acid nanoparticles; DNA nanotechnology; DNA Synthesis; DNA amplification 1. Introduction In all known living organisms, DNA molecules are responsible for storing and carrying genetic information [1]. From a materials and biomedical engineering point of view, DNA molecules also represent a promising alternative to several natural and synthetic polymers that are typically used for biomedical applications such as drug delivery and cancer immunotherapy [2–6]. DNA-based materials differ from other polymeric materials as they offer programmability at the nanoscale, along with unique structural and biochemical properties [7,8]. These characteristics make them ideal as building blocks to assemble complex nanoarchitectures and further organize various biomolecules and inorganic molecules with nanometer-scale precision [7–11]. In vivo, DNA molecules are primarily present as B-form double-stranded DNA (dsDNA) molecules, comprising two complementary single strands of DNA (ssDNA) assembled by hybridization through Watson–Crick base pairing [12]. They exhibit a right-handed double-helix structure (2 nm diameter) with a periodicity of 10.5 bases and a distance of 3.4 Å between each base pair [1,12]. Leveraging the sequence specificity and unique structural features of dsDNA along with the structural predictability of DNA assembly has facilitated the rapid Molecules 2020, 25, 3386; doi:10.3390/molecules25153386 www.mdpi.com/journal/molecules Molecules 2020, 25, 3386 2 of 18 development of the structural DNA nanotechnology field [11,13–15], and particularly enabled the emergence ofMolecules the 2020 DNA, 25, x FOR origami PEER REVIEW method [16]. This latter method rapidly became2 of the 18 strategy of choice for synthesizing discrete nanometer-scale particles, notably enabling the assembly of custom of DNA assembly has facilitated the rapid development of the structural DNA nanotechnology field complex 1-, 2-,[11,13–15], and 3D and discrete particularly DNA enabled nanoarchitectures the emergence of the with DNA highly origami defined method [16]. shapes This andlatter sizes [16–20]. DNA origamimethod nanoparticles rapidly became are the assembled strategy of choice by folding for synthesizing a long discrete ssDNA nanometer-scale scaffold strand particles, with an excess of several shortnotably complementary enabling the assembly oligonucleotides of custom complex 1-, (‘staple 2-, and 3D strands’) discrete DNA in nanoarchitectures a one-pot thermal with annealing highly defined shapes and sizes [16–20]. DNA origami nanoparticles are assembled by folding a long reaction (FiguressDNA1a). scaffold These strand nanoparticles with an excess are nowof severa widelyl short used complementary in many biomedicaloligonucleotides applications, (‘staple such as nucleic acid deliverystrands’) in [ 20a one-pot,21], vaccine thermal annealing platform reaction development (Figure 1a). [These22–24 nanoparticles], drug delivery are now[ widely25–27 ], and cancer therapy [28–31used], amongin many othersbiomedical [32 applications,–34]. such as nucleic acid delivery [20,21], vaccine platform development [22–24], drug delivery [25–27], and cancer therapy [28–31], among others [32–34]. Figure 1. DNAFigure origami 1. DNA nanoparticle origami nanoparticle assembly. assembly. (a) DNA(a) DNA origami origami areare folded with with a long a long single- single-stranded stranded DNA scaffold and multiple staple strands via a thermal annealing process. (b) Controlling DNA scaffoldthe and DNA multiple origami size staple with custom strands scaffold via lengths. a thermal annealing process. (b) Controlling the DNA origami size with custom scaffold lengths. Unlike other nucleic-acid-based nanoparticle assembly techniques that rely on the equimolar Unlike otherassembly nucleic-acid-based of short oligonucleotides nanoparticle [35,36], the complexity assembly and size techniques of the assembled that DNA rely origami on the equimolar nanostructures mainly depend on the scaffold strand length, sequence, and method of production assembly of[37] short (Figure oligonucleotides 1b). Furthermore, the [35 amount,36],the of DNA complexity origami nanoparticles and size that of can the be assembled assembled will DNA origami nanostructuresdepend mainly on the depend scaffold availability. on the sca DNAffold origam strandi nanoparticles length, sequence, are commonly and assembled method using of the production [37] (Figure1b). Furthermore,M13mp18 bacteriophage’s the amount genome—a of DNAcommercially origami available nanoparticles 7249 nucleotide that (nt)-long can becircular assembled will single strand of DNA—which can readily be used to assemble nanoparticles in a 10 to 100 nm size depend on therange sca [16–19,38].ffold availability. However, the DNAemergence origami of several nanoparticles new types of design are commonly software [19,39–42] assembled using the M13mp18enabling bacteriophage’s the automated design genome—a of complex commercially nanostructures with available any shape 7249or size—and nucleotide the increasing (nt)-long circular single strandnumber of DNA—which of biomedical applications can readily [43–45] have be usedled to the to increased assemble complexity nanoparticles and size of designed in a 10 to 100 nm DNA origami. The standard M13mp18 scaffold strand may limit the sizes of these newly designed size range [16nanoarchitectures.–19,38]. However, In addition, the the emergence sequence of the of scaffold several might new affect types the performance of design of the software DNA [19,39–42] enabling theorigami automated nanostructures design for of a complexgiven application. nanostructures For instance, withrecent anyworks shape suggestor the size—and importance of the increasing number of biomedicalsequence design applications to ensure immunocompatibility [43–45] have led of the to DNA the nanoparticles increased complexity[46]. Thus, controlling and size the of designed sequence of the scaffold strands to avoid the presence of phage genes that might have an undesirable DNA origami.effect The for in standard vivo applications, M13mp18 or controlling scaffold the presence strand or may absence limit of immunogenic the sizes of CpG these domains newly designed nanoarchitectures.is necessary. Inaddition, For all these the reasons, sequence the use of M13mp18 the scaff ssDNAold might as the sole affect source the of performance scaffolding for of the DNA origami nanostructuresDNA origami synthesis for a given is now application. becoming a limiting For instance, factor. To design recent custom works nanostructures suggest the and importance of precisely control their sequences, establishing novel and efficient custom ssDNA scaffold synthesis sequence designmethods to ensureis crucial immunocompatibility to leverage DNA origami’s of full the potential. DNA nanoparticlesTo be sustainable [ 46for]. biomedical Thus, controlling the sequence of theapplications, scaffold the strands production to scale avoid of ssDNA the presence scaffolds also of needs phage to be genes drastically that increased might haveto reduce an undesirable effect for in vivothe costsapplications, of production orand controlling enable a broader the range presence of applications. or absence of immunogenic CpG domains is This review focuses on existing and emerging techniques for the synthesis of ssDNA scaffolds necessary. Forfor allDNA these origami reasons, folding. theIn particular, use of M13mp18
Details
-
File Typepdf
-
Upload Time-
-
Content LanguagesEnglish
-
Upload UserAnonymous/Not logged-in
-
File Pages18 Page
-
File Size-