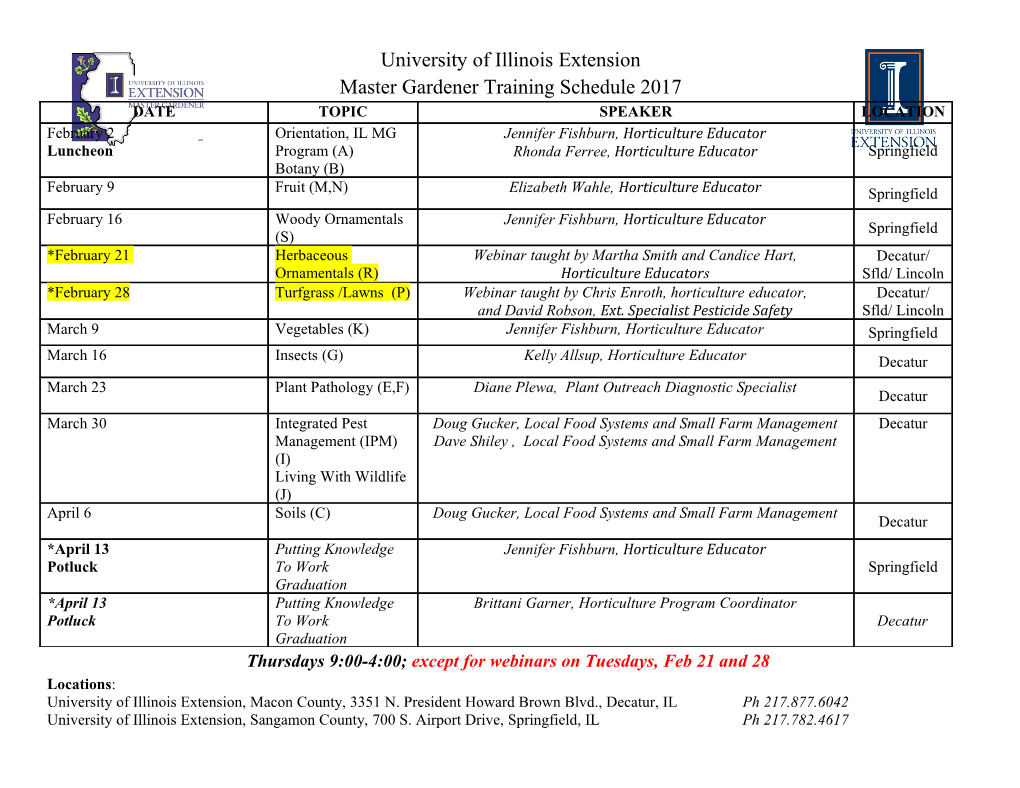
Eur. Phys. J. Special Topics 226, 1623–1694 (2017) © The Author(s) 2017 THE EUROPEAN DOI: 10.1140/epjst/e2017-70071-y PHYSICAL JOURNAL SPECIAL TOPICS Regular Article The electron capture in 163Ho experiment – ECHo L. Gastaldo1,a,K.Blaum2, K. Chrysalidis3,T.DayGoodacre4,A.Domula5, M. Door2,H.Dorrer6,7,8,Ch.E.D¨ullmann6,9,10,K.Eberhardt6,10, S. Eliseev2, C. Enss1, A. Faessler11, P. Filianin2, A. Fleischmann1, D. Fonnesu1, L. Gamer1, R. Haas6, C. Hassel1, D. Hengstler1, J. Jochum12,K.Johnston4, U. Kebschull13, S. Kempf1,T.Kieck3,6,U.K¨oster14,S.Lahiri15, M. Maiti16, F. Mantegazzini1, B. Marsh4, P. Neroutsos13, Yu.N. Novikov2,17,18, P.C.O. Ranitzsch1,b, S. Rothe4, A. Rischka2,A.Saenz19, O. Sander20, F. Schneider3,6, S. Scholl12,R.X.Sch¨ussler2, Ch. Schweiger2, F. Simkovic21,T.Stora4,Z.Sz¨ucs22,A.T¨urler7,8, M. Veinhard4, M. Weber20, M. Wegner1, K. Wendt3, and K. Zuber5 1 Kirchhoff Institute for Physics, Heidelberg University, Heidelberg, Germany 2 Max-Planck Institute for Nuclear Physics, Heidelberg, Germany 3 Institute for Physics, Johannes Gutenberg-University, Mainz, Germany 4 ISOLDE, CERN, Geneve, Switzerland 5 Institute for Nuclear and Particle Physics, TU Dresden, Germany 6 Institute for Nuclear Chemistry, Johannes Gutenberg University, Mainz, Germany 7 Laboratory of Radiochemistry and Environmental Chemistry, Department Biology and Chemistry, Paul Scherrer Institute, Villigen PSI, Switzerland 8 Laboratory of Radiochemistry and Environmental Chemistry, Department of Chemistry and Biochemistry, University of Bern, Freiestrasse 3, Bern, Switzerland 9 GSI Helmholtzzentrum f¨ur Schwerionenforschung, Darmstadt, Germany 10 Helmholtz Institute Mainz, Mainz, Germany 11 Institute for Theoretical Physics, University of T¨ubingen,T¨ubingen,Germany 12 Physics Institute, University of T¨ubingen,Germany 13 Goethe-Universit¨at, Frankfurt am Main, Germany 14 Institut Laue-Langevin, Grenoble, France 15 Chemical Sciences Division, Saha Institute of Nuclear Physics, 1/AF Bidhannagar, Kolkata, India 16 Department of Physics, Indian Institute of Technology Roorkee, Roorkee, India 17 Petersburg Nuclear Physics Institute, Gatchina, Russia 18 St.Petersburg State University, St. Petersburg, Russia 19 Institut f¨ur Physik, Humboldt-Universit¨atzu Berlin, Berlin, Germany 20 Karlsruhe Institute of Technology, Institute for Data Processing and Electronics, Karlsruhe, Germany 21 Department of Nuclear Physics and Biophysics, Comenius University, Bratislava, Slovakia 22 Institute of Nuclear Research of the H.A.S., Bem ter 18/C, Debrecen, Hungary Received 17 March 2017 Published online 20 June 2017 a e-mail: [email protected] b Present address: Institut f¨urKernphysik, Universit¨at M¨unster,M¨unster, Germany 1624 The European Physical Journal Special Topics Abstract. Neutrinos, and in particular their tiny but non-vanishing masses, can be considered one of the doors towards physics beyond the Standard Model. Precision measurements of the kinematics of weak interactions, in particular of the 3H β-decay and the 163Ho electron capture (EC), represent the only model independent approach to de- termine the absolute scale of neutrino masses. The electron capture in 163Ho experiment, ECHo, is designed to reach sub-eV sensitivity on the electron neutrino mass by means of the analysis of the calorimet- rically measured electron capture spectrum of the nuclide 163Ho. The maximum energy available for this decay, about 2.8 keV, constrains the type of detectors that can be used. Arrays of low temperature metal- lic magnetic calorimeters (MMCs) are being developed to measure the 163Ho EC spectrum with energy resolution below 3 eV FWHM and with a time resolution below 1 μs. To achieve the sub-eV sensitivity on the electron neutrino mass, together with the detector optimization, the availability of large ultra-pure 163Ho samples, the identification and suppression of background sources as well as the precise parame- trization of the 163Ho EC spectrum are of utmost importance. The high-energy resolution 163Ho spectra measured with the first MMC prototypes with ion-implanted 163Ho set the basis for the ECHo exper- iment. We describe the conceptual design of ECHo and motivate the strategies we have adopted to carry on the present medium scale exper- iment, ECHo-1K. In this experiment, the use of 1 kBq 163Ho will allow to reach a neutrino mass sensitivity below 10 eV/c2. We then discuss how the results being achieved in ECHo-1k will guide the design of the next stage of the ECHo experiment, ECHo-1M, where a source of the order of 1 MBq 163Ho embedded in large MMCs arrays will allow to reach sub-eV sensitivity on the electron neutrino mass. 1 Introduction Although the current Standard Model (SM) is a very successful and accurate de- scription of particle physics, we know it is incomplete and an extension is needed to understand the structure of the universe, the hierarchy of masses and to achieve a grand unification. At the Large Hadron Collider (LHC) so far experimental hints to physics beyond the SM are missing and the favored supersymmetric extension of the SM seems to be less likely. The observation of neutrino oscillations [1,2]about20 years ago, establishing that neutrinos must be massive, gives a clear hint of physics beyond the SM. To guide the development of theories beyond the SM, one of the most interesting open questions is therefore the absolute scale of neutrino masses. From neutrino oscillations, only the differences of the masses squared can be ex- tracted [3], but not the absolute values. The determination of the neutrino mass scale is a challenging task due to the smallness of the masses and to the weak interaction with other standard model particles. There exist several approaches which can po- tentially yield this result: the analysis of the visible structures in the universe [4], the observation of neutrinoless double beta decay [5,6] and resonantly enhanced double electron capture [7], the measurement of the time of flight of neutrinos emitted in supernova explosions [8] and the analysis of the kinematics of low energy beta decays and electron capture (EC) processes [9]. In particular, direct kinematical methods allow to perform a model-independent measurement of the electron neutrino and an- tineutrino mass with high sensitivity and low systematic uncertainties. In the past this approach was mainly driven by the investigation of the 3H beta spectrum. KATRIN, the KArlsruhe TRItium Neutrino experiment [10], is designed to either measure the electron-antineutrino mass down to 0.3 eV/c2 or alternatively give an upper limit of The Electron Capture in 163Ho Experiment – ECHo 1625 0.2 eV/c2 with 95% C.L. [9]. These values are approximately a factor of ten smaller than the 95% C.L. upper limits of 2.12 and 2.30 eV/c2 that the two precursor experiments, “Troitsk neutrino mass experiment” and “The Mainz Neutrino mass experiment” respectively, determined [11–13]. Together with KATRIN, two other ex- periments are designed to improve the limit on the electron anti-neutrino mass ana- lyzing the 3H beta-spectrum, these are Project8 [14] and PTOLEMY [15]. The most 2 stringent limit for the electron neutrino mass is mνe < 225 eV/c (95% C.L.) obtained by the analysis of the Internal Bremsstrahlung in Electron Capture (IBEC) spec- trum of 163Ho [16]. The Electron Capture in 163Ho experiment, ECHo, is designed to investigate the electron neutrino mass in the sub-eV region by the analysis of the calorimetrically measured electron capture spectrum of 163Ho. 2 Electron capture in 163Ho and neutrino mass Given the present knowledge, 163Ho is the best candidate to perform an experiment 163 to investigate the neutrino mass in the sub-eV region. The half-life of Ho is T1/2 = 4570 ± 50 y [17,18]. According to the last release of the Atomic-Mass Evaluation by Audi et al. [19], the recommended value for the energy available to the decay 163 of Ho is QEC =2.555 ± 0.016 keV obtained by combining several measurements whose results actually span over a larger range, from QEC =2.3 ± 1 keV [20]to QEC 2.8 keV [21,22]. In all these measurements the QEC-value was determined by the analysis of the energy spectrum of 163Ho, measured with different techniques. In order to remove any systematic error in the interpretation of the measured spectra, which could have led to the large discrepancies, the ECHo collaboration has recently determined the energy available to the decay of 163Ho by directly measuring the mass difference between 163Ho and 163Dy using the Penning-trap mass spectrometry technique (PTMS). The result is QEC =2.833 ± 0.030stat ± 0.015syst keV [23]. The experimental technique and the challenges to reduce the uncertainties on the QEC- value to 1 eV will be described in Section 9. 163 P − 163 P In the EC process, a 67Ho nucleus (J =7/2 ) decays to a 66Dy nucleus (J = 5/2−) by capturing an electron from an inner atomic shell and emitting an electron neutrino. A tiny fraction of energy, less than 1 meV, is taken by the nuclear recoil due to the emission of the neutrino. The rest of the energy is shared between the emitted electron neutrino and the atomic excitations of the daughter atom 163Dy. The small QEC-value energetically limits the capture processes to electrons occupying the third and higher shells. Among those, only electrons from s-levels and p1/2-levels can be captured due to angular momentum conservation and to the finite overlap of their wave function with the nucleus. The excited state in which the daughter atom 163Dy is left after the EC in 163Ho is characterized, for first order transitions, by a hole in an inner shell, corresponding to the captured electron, and an additional electron in the 4f-shell. Higher order excited states with more than one hole in the atomic shells, due to shake-up or shake- off processes, have a much smaller probability to be created [24].
Details
-
File Typepdf
-
Upload Time-
-
Content LanguagesEnglish
-
Upload UserAnonymous/Not logged-in
-
File Pages72 Page
-
File Size-