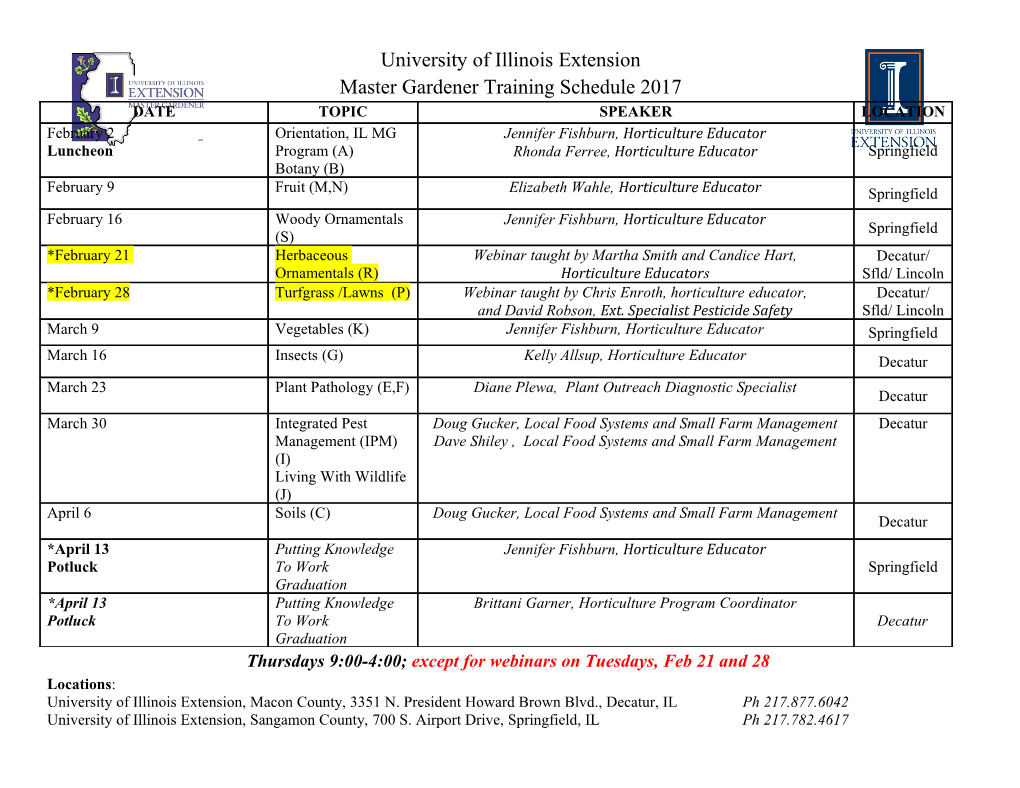
ARTICLE IN PRESS Mapping Protein–Ligand Interactions with Proteolytic Fragmentation, Hydrogen/ Deuterium Exchange-Mass Spectrometry Elyssia S. Gallagher*,†,1, Jeffrey W. Hudgens*,†,1 *Institute for Bioscience and Biotechnology Research, Rockville, Maryland, USA †Biomolecular Measurement Division, National Institute of Standards and Technology, Rockville, Maryland, USA 1Corresponding authors: e-mail address: [email protected]; [email protected] Contents 1. Introduction 2 2. H/D Exchange Theory 3 2.1 H/D Exchange 3 2.2 Acid and Base Catalysis 5 2.3 Temperature 6 2.4 Protein Structure 6 2.5 Effects of Ligand-Binding Interactions upon H/D Exchange Rates 8 3. The HDX-MS Experiment 12 3.1 Synopsis 12 3.2 Protein Preparation 13 3.3 Exchange Reaction 15 3.4 Automated Versus Manual HDX-MS 16 3.5 Reaction Quenching 17 3.6 Enzymatic Digestion 18 3.7 Chromatography 19 3.8 Mass Spectrometry 20 3.9 Generating a Proteomic Map 27 3.10 Data Analysis 28 3.11 Uncertainty Evaluations: Which Deuterium-Uptake Differences Are Meaningful? 30 3.12 Data Display 31 1 Current address: Department of Chemistry and Biochemistry, Baylor University, Waco, Texas, USA. # Methods in Enzymology 2015 Elsevier Inc. 1 ISSN 0076-6879 All rights reserved. http://dx.doi.org/10.1016/bs.mie.2015.08.010 ARTICLE IN PRESS 2 Elyssia S. Gallagher and Jeffrey W. Hudgens 4. Interpreting HDX-MS Data to Determine Protein–Ligand Interaction Maps 32 4.1 Example: Protein–Ligand Interactions Involving Continuous Amide Contacts 32 4.2 Example: Mapping a Discontinuous Protein–Protein Interaction 37 Disclaimer 39 References 41 Abstract Biological processes are the result of noncovalent, protein–ligand interactions, where the ligands range from small organic and inorganic molecules to lipids, nucleic acids, peptides, and proteins. Amide groups within proteins constantly exchange protons with water. When immersed in heavy water (D2O), mass spectrometry (MS) can measure the change of mass associated with the hydrogen to deuterium exchange (HDX). Protein–ligand interactions modify the hydrogen exchange rates of amide protons, and the measurement of the amide exchange rates can provide rich information regard- ing the dynamical structure of the protein–ligand complex. This chapter describes a pro- tocol for conducting bottom-up, continuous uptake, proteolytic fragmentation HDX-MS experiments that can help identify and map the interacting peptides of a protein–ligand interface. This tutorial outlines the fundamental theory governing hydrogen exchange; provides practical information regarding the preparation of protein samples and solu- tions; and describes the exchange reaction, reaction quenching, enzymatic digestion, chromatographic separation, and peptide analysis by MS. Tables list representative combinations of fluidic components used by HDX-MS researchers and summarize the available HDX-MS analysis software packages. Additionally, two HDX-MS case stud- ies are used to illustrate protein–ligand interactions involving: (1) a continuous sequence of interacting residues and (2) a set of discontinuously numbered residues, residing spatially near each other. 1. INTRODUCTION Biological processes are facilitated by noncovalent, protein–ligand interactions. Ligands range from small organic and inorganic molecules to lipids, nucleic acids, peptides, and proteins. As examples, in the respiration cycle reversible interactions of O2 and carbamino compounds at distinct sites within hemoglobin enable transport of O2 and CO2 (Nelson & Cox, 2012). In blood plasma, progesterone, propranolol, and other small therapeutic molecules are carried by α1-acid glycoprotein (Fournier, Medjoubi-N, & Porquet, 2000; Huang & Hudgens, 2013). Additionally, a plethora of small molecule–protein (Kirschke, Goswami, Southworth, Griffin, & Agard, 2014), peptide–protein (Artimo et al., 2012; Das, Sharma, Kumar, Krishna, & Mathur, 2013), and protein–protein interactions mediate signal ARTICLE IN PRESS Mapping Protein–Ligand Interactions with HDX-MS 3 transduction across the plasma membrane and within living cells (Chen, Chattopadhyay, Bergen, Gadd, & Tannery, 2007). Hydrogen/deuterium exchange-mass spectrometry (HDX-MS) is an important tool for characterizing protein–ligand interactions (Katta & Chait, 1991; Zhang & Smith, 1993). HDX-MS measures deuterium uptake of amide N–H groups along the protein backbone. This information is use- ful for identifying portions of the protein engaged in binding and the dynamics of the protein–ligand interface. While once quite challenging and labor intensive, advances in instrumentation and software have enabled research groups to rapidly complete insightful, conclusive HDX-MS studies. As a result, more laboratories are conducting analyses that utilize HDX-MS methods (Pirrone, Iacob, & Engen, 2015). This chapter describes protocols for conducting studies with continuous uptake, proteolytic fragmentation HDX-MS, which has demonstrated utility for identifying the interacting peptides in protein–ligand interactions. The power of this HDX-MS protocol for mapping protein–protein interactions is demonstrated in a study by Malito et al. (2013), who used multiple tech- niques to map the epitope of complement factor H-binding protein (fHbp) against a monoclonal antibody (mAb 12C1). Malito et al. studied the inter- action of fHbp with bactericidal mAb 12C1 with diverse mapping techniques, including peptide arrays, phage display, X-ray crystallography, and HDX-MS (Fig. 1A–D). The map obtained with each technique was compared to the epitope of fHbp (Fig. 1E), determined from modeling the fHbp:fH crystal structure (Schneider et al., 2009). Their HDX-MS study accounted for nearly the entire 1000 A˚ 2 contact interface between mAb 12C1 and fHbp. They concluded that “…hydrogen/deuterium exchange- mass spectrometry was the most effective method to rapidly supply near- complete information about epitope structure” (Malito et al., 2013). 2. H/D EXCHANGE THEORY 2.1 H/D Exchange Within proteins, hydrogen atoms manifesting ionic character exchange with the labile protons of the surrounding solvent. When the solvent contains an isotope of hydrogen (deuterium or tritium), exchange can be used to char- acterize protein structure and dynamics via an analytical method that detects a difference between the isotopes. Since hydrogen and deuterium have masses of 1.008 and 2.014 Da, respectively, MS can be utilized to monitor exchange by detecting the mass increase of the protein. ARTICLE IN PRESS 4 Elyssia S. Gallagher and Jeffrey W. Hudgens Figure 1 Binding maps of the fHbp antigenic epitope plotted by different techniques (Malito et al., 2013). The N- and C-terminal domains of fHbp are presented in green (light gray in the print version) and cyan (light gray in the print version), respectively. (A) Red (dark gray in the print version) denotes the fHbp epitope found by synthetic peptide arrays. (B) Red (dark gray in the print version) denotes the fHbp epitope found by phage display. (C) Red (dark gray in the print version) denotes the 23 residues of fHbp con- tacted by the Fab of mAb 12C1, as determined from the X-ray structure of the fHbp: Fab 12C1 complex. (D) Red (dark gray in the print version) denotes the peptide regions of fHbp contacted by the Fab of mAb 12C1 within the fHbp:12C1 complex, as deter- mined by HDX-MS measurements. (E) Red (dark gray in the print version) denotes 52 fHbp surface residues bound with surface residues of domains 6 and 7 in human fH, as prepared by Malito et al. by modeling the crystal structure of fHbp:fH complex (Schneider et al., 2009). Adapted images used with the permission of Malito et al. (2013). Each hydrogen bond type has a distinct range of H/D exchange rates (Fig. 2). For instance, covalently bound C–H groups do not undergo solvent-mediated exchange. Covalently bound hydrogen atoms in amine, indole, and hydroxyl functional groups of amino acid side chains and the N-terminal amide group of the protein backbone undergo rapid H/D exchange with half-lives on the order of t1/2 0.01–1 ms (Bai, Milne, Mayne, & Englander, 1993; Liepinsh & Otting, 1996). The other amides in the protein backbone exhibit exchange rates with half-lives ranging from t1/2 ¼5 s to 60 days. These rates can be measured using proteolytic fragmen- tation HDX-MS. Since a backbone amide hydrogen is present at every amino acid (except proline) throughout the protein, monitoring the rates of exchange associated with these functional groups allows the entire protein structure to be sampled. In addition to the functional group, H/D exchange rates vary with solution pH, temperature, solvent accessibility, and protein structure (Balasubramaniam & Komives, 2013; Englander & Kallenbach, 1984; Mandell, Falick, & Komives, 1998). ARTICLE IN PRESS Mapping Protein–Ligand Interactions with HDX-MS 5 H O H O S H H H CH3 O 2 O 2 O H H + H N H N H N H N N N O H H H 2 H H H H H O O H2 H H2 O H2 N H N H 2 H H 2 + H N H H Gly – Asp – His – Cys – Lys – Ala Figure 2 Hydrogens classified by exchangeability in an example peptide: glycine- aspartic acid-histidine-cysteine-lysine-alanine. HDX-MS measures the backbone amide hydrogens shown in green (light gray in the print version) pentagons. Hydrogens that are not monitored with HDX-MS due to their very rapid exchange rates are shown in red (dark gray in the print version) circles. The numerous hydrogens that do not exchange due to their covalent binding with carbon are designated by black letters. 2.2 Acid and Base Catalysis A completely unfolded protein has essentially no protection against proton exchange between the solvent and each amide group of the protein back- bone, that is, each amide is in the exchange-competent state, N–Hopen. Pro- ton exchange between an unprotected amide group and aqueous solvent is a chemical reaction catalyzed by acid, base, or water. Accordingly, the exchange rate (kch) is written (Bai et al., 1993; Englander, 2006; Englander & Kallenbach, 1984): int + int À int kch ¼ kacid½H3O + kbase½OH + kwater½H2O (1) int int The kacid and kbase are rate coefficients for the acid- and base-catalyzed int exchange, and kwater is the intrinsic rate coefficient for the water-catalyzed exchange reaction (Englander, 2006).
Details
-
File Typepdf
-
Upload Time-
-
Content LanguagesEnglish
-
Upload UserAnonymous/Not logged-in
-
File Pages48 Page
-
File Size-