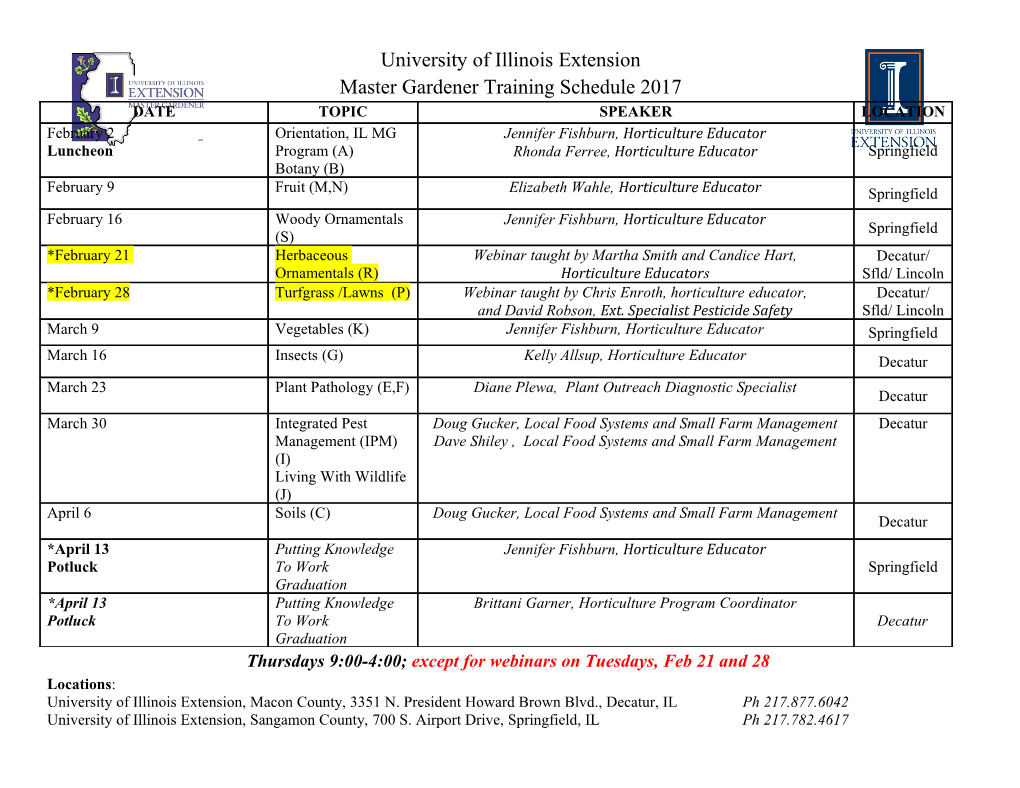
Cuttlebone: Characterization and Applications By: Safieh Momeni Cuttlebone Cuttlebone signifies a special class of ultra-lightweight, high stiffness and high permeability cellular biomaterials, providing the cuttlefish with an efficient means of maintaining neutral buoyancy at considerable habitation depths. In addition, this rigid cellular material provides the structural backbone of the body and plays a key role in the protection of vital organs. Cuttlebone The cuttlebone has two main components: Dorsal shield Lamellar matrix The dorsal shield is very tough and dense, providing a rigid substrate for protection, structure and the development of the lamellar matrix of cuttlebone. The lamellar matrix of cuttlebone has an extreme porosity (up to 90%), but also manages to withstand very high hydrostatic pressure. Lamellar matrix The lamellar matrix consists primarily of aragonite (a crystallised form of calcium carbonate, CaCO3), enveloped in a layer of organic material composed primary of β-chitin. The organic layer entirely envelopes the inorganic ceramic, and is thought to initiate, organise and inhibit the mineralisation of the inorganic material. From a mechanical perspective, the organic layer is also thought to provide a certain toughening effect to the material Applications Due to the unique physical, chemical and mechanical characteristics of this natural cellular material, a range of novel applications for the material have recently been investigated. Preparation of highly porous hydroxyapatite from cuttlefish bone Hydroxyapatite structures for tissue engineering applications have been produced by hydrothermal (HT) treatment of aragonite in the form of cuttlefish bone at 200 ̊C. Aragonite (CaCO3) monoliths were completely transformed into hydroxyapatite after 48 h of HT treatment. 2- 3- The substitution of CO3 groups predominantly into the PO4 sites of the Ca10(PO4)6(OH)2 structure was suggested by FT-IR spectroscopy and Rietveld structure refinement. The SEM micrographs have shown that the interconnected hollow structure with pillars connecting parallel lamellae in cuttlefish bone is maintained after conversion. Specific surface area (SBET) increased and mean pore size decreased by HT treatment. J Mater Sci: Mater Med (2009) 20:1039–1046 Hydroxyapatite Hydroxyapatite (HAP; Ca10(PO4)6(OH)2) due to its similarity with the mineral phase of bone has been studied for many years as an implant material . In recent years, particular attention has been paid to the synthesis of HAP ceramics with the porous morphology required for vascularization, bone cell invasion, and angiogenesis, which further improve its biomedical properties. The HAP structure can incorporate a wide variety of ions implying a variable defect structure of HAP. In biological apatites, or apatites originating from biogenetic materials, the presence of other mineral ions, such as Na+,K+,Mg2+, F- as well as substitution of carbonate ions into the HAP structure is very common. The carbonate ion substitutes either at the phosphate tetrahedron (B-type substitution) or at the hydroxyl site (A-type substitution) . The biological apatite that constitutes bone mineral is considered to be of mixed AB-type substitution . Hydroxyapatite While synthetic materials have been widely used in the biomedical field with great success, natural structural materials are now providing an abundant source of novel biomedical applications. During the last decade, an increased understanding of biomineralization has initiated improvements in biomimetic synthesis methods and production of new generation of biomaterials. The use of natural biogenic structures and materials such as corals, seashells, animal bones, etc., for medical purposes has been motivated by limitations in generating synthetic materials with the requisite structure and mechanical integrity. Hydroxyapatite Dry cuttlefish bone was cut into small pieces about 2 cm3 large and was first heated to 350 ºC for 3 h to remove the organic component, and then sealed with the 15 ml aqueous solution of 0.6 M NH4H2PO4 in Teflon lined stainless steel pressure vessel at 200ºC for various times (1–48 h). The stoichiometrically required aqueous solution of NH4H2PO4 to set the ratio Ca/P = 1.67 was determined using the data of DSC and TG analysis of raw cuttlefish bones. The formed HAP was washed with boiling water and dried at 110ºC. SEM micrographs of: a cuttlefish bone heated at 350C for 3 h. The corrugated appearance of the pillars is shown in the inset, b the cuttlefish bone after hydrothermal conversion at 200 C/24 h showing plate- and needle-like HAP crystals (inset) Biotemplated Syntheses of Macroporous Materials for Bone Tissue Engineering Scaffolds and Experiments in Vitro and Vivo ACS Appl. Mater. Interfaces The macroporous materials were prepared from the transformation of cuttlebone as biotemplates under hydrothermal reactions. Cell experimental results showed that the prepared materials as bone tissue engineering scaffolds or fillers had fine biocompatibility suitable for adhesion and proliferation of the hMSCs (human marrow mesenchymal stem cells). Histological analyses were carried out by implanting the scaffolds into a rabbit femur, where the bioresorption, degradation, and biological activity of the scaffolds were observed in the animal body. The prepared scaffolds kept the original three-dimensional frameworks with the ordered porous structures, which made for blood circulation, nutrition supply, and the cells implantation. The biotemplated syntheses could provide a new effective approach to prepare the bone tissue engineering scaffold materials. Design and synthesis of new porous scaffold materials for bone rehabilitation are of great significance since large quantities of skeletal reconstructive surgical cases need to be performed with the scaffold materials each year worldwide. In bone healing occurrence, the graft materials play the crucial roles.The autologous bones, as the gold-standard of the graft materials, can provide the scaffolds and active factors for bone ingrowth. However, aside from the source of the autologous bone being greatly limited, the autologous bone grafts are associated with an 8−39% risk of complications, e.g., hematoma, additional injury, superinfection, surgical complication, postoperative pain, and chronic pain at the donor sites. Therefore, autologous bone grafts are normally not recommended for elderly or pediatric patients or for patients with malignant or infectious disease. Alternative strategies, like allo- or xeno-transplantations, have major biocompatibility disadvantages compared with autografts. To overcome these limitations, the bone graft substitutes have been used to reconstruct bone defects. The perfect bone substitutes are osteoinductive, osteoconductive, biocompatible, and bioresorbable, which should induce minimal or no grafts rejection and can undergo remodeling and support new bone formation. On the other hand, it should be cost-effective and available in the amount required. The bone substitutes as scaffolds are in favor of the bone cells migration, proliferation, and new bone formation. Calcium phosphate-based ceramics are currently available and widely used in trauma and orthopedic surgery for bone substitutes due to their chemical similarity to bone mineral with minimal immunologic reactions, no foreign body reactions, or no systemic toxicity. Hydroxyapatite (HA, Ca5(PO4)3(OH)) and beta tricalciumphosphate (β-TCP, Ca3(PO4)2) are well-known bioceramics that possess high tissue compatibility and osteoconductivity. However, HA seems to be too stable in vivo because it shows a similar crystalline phase as bone mineral, which would be hard to tend toward chemical and biological equilibrium with bone tissue. Preparation of the Sca old Materials Cuttlebones (CB, 100 g, 30 × 10 × 3.5 cm) fromffthe sampan-like spine of cuttlefishes were cut into di erent dimensional cylinders. The mixture of pieces of dry cuttlebone and (NH4)2HPOff4 (mol/mol, 1:1.1) in aqueous solution were sealed in a 100 mL stainless steel autoclave with Teflon liner and placed in a temperature-controlled electric furnace at 180 °C (heating and cooling rates were 10 K·min−1). Di erent times of hydrothermal reaction were tested between 3 and 48 h in independentffexperiments. The pH of the solution was 7.8−8.2 before being placed in autoclaves and slightly lower after the hydrothermal reaction. FTIR spectra of treated cuttlebone (a) and the scaffold transformed from hydrothermal reaction at 180 °C for 3 h (b), 6 h (c), 12 h (d), 24 h (e), and 48 h (f). XRD patterns. Aragonite (ICDD card 01-071-2396) (a), cuttlebone (b), hydroxyapatite (ICDD card 00- 009-0432) (c), and hydrothermal reaction for 3 h (d), 6 h (e), 12 h (f), and 24 h (g). TG-DTA curves. (a) Cuttlebones; (b) scaffold materials transformed from cuttlebones by hydrothermal reactions. (a) Scanning microscope view of the sintered scaffold transformed from the cuttlebone by hydrothermal reaction, and all the trabeculares were broken in the holes; (b) original magnification of 400× clearly reveals the ordered macroporous framework supported by the S-shaped pillars; (c) the floor supported by the “S” oriented walls; (d) transverse section view of the magnification of 10 000× of the floor with 2−5 μm openings; (e) image of exterior surface of the floor wall; (f) view of the magnification of 30 000× for the ceramic floor. (a) Top view of the scaffold; (b) the top view of the “S” oriented wall; (c) the nanocrystallite composites at the
Details
-
File Typepdf
-
Upload Time-
-
Content LanguagesEnglish
-
Upload UserAnonymous/Not logged-in
-
File Pages64 Page
-
File Size-