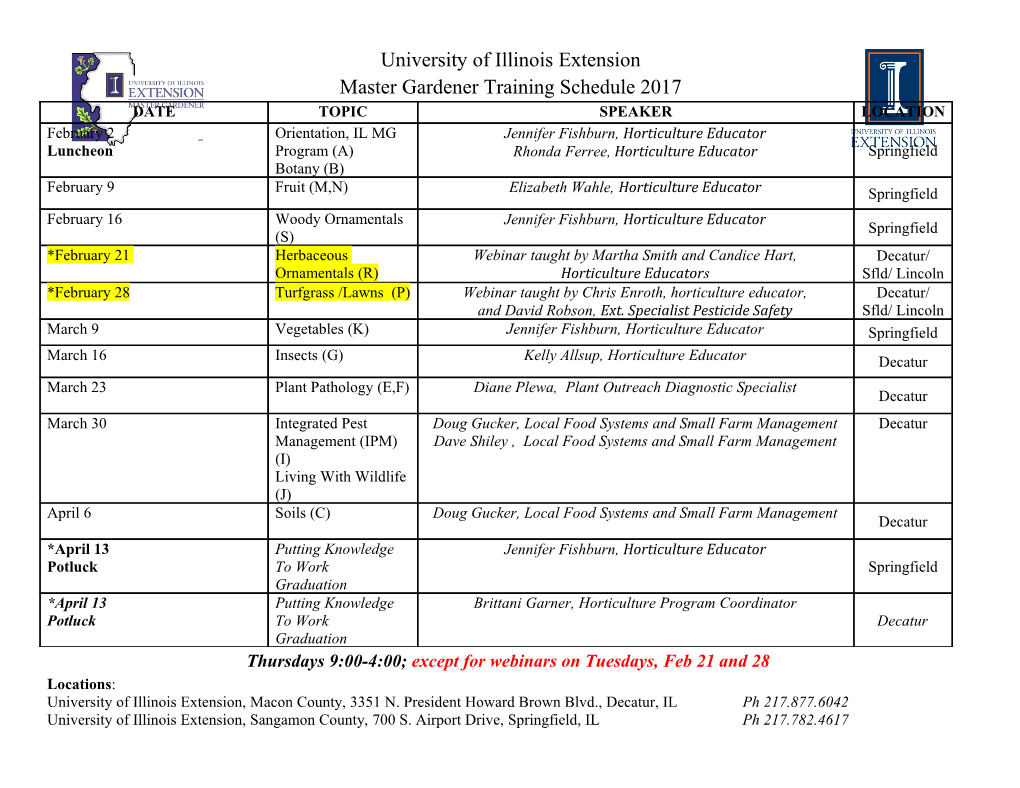
“One-Pot” Oligomeric A 2 + B 3 Approach to Branched Poly(arylene ether sulfone)s: Reactivity Ratio Controlled Polycondensation A thesis submitted in partial fulfillment of the requirements for the degree of Master of Science By ANDREA M. ELSEN B.S., Wright State University, 2007 2009 Wright State University WRIGHT STATE UNIVERSITY SCHOOL OF GRADUATE STUDIES June 19, 200 9 I HEREBY RECOMMEND THAT THE THESIS PREPARED UNDER MY SUPERVISION BY Andrea M. Elsen ENTITLED “One-Pot” Oligomeric A2 + B3 Approach to Branched Poly(arylene ether sulfone)s: Reactivity Ratio Controlled Polycondenstation BE ACCEPTED IN PARTIAL FULFILLMENT OF THE REQUIREMENTS FOR THE DEGREE OF Master of Science . _________________________ Eric Fossum, Ph.D. Thesis Director _________________________ Kenneth Turnbull, Ph.D. Department Chair Committee on Final Examination ____________________________ Eric Fossum, Ph.D. ____________________________ Kenneth Turnbull, Ph.D. ____________________________ William A. Feld, Ph.D. ____________________________ Joseph F. Thomas, Jr., Ph.D. Dean, School of Graduate Studies Abstract Elsen, Andrea M. M.S., Department of Chemistry, Wright State University, 2009. “One-Pot” Oligomeric A 2 + B 3 Approach to Branched Poly(arylene ether sulfone)s: Reactivity Ratio Controlled Polycondensation The synthesis of fully soluble branched poly(arylene ether)s via an oligomeric A 2 + B 3 system, in which the A 2 oligomers are generated in situ, is presented. This approach takes advantage of the significantly higher reactivity toward nucleophilic aromatic substitution reactions, NAS, of B 2, 4-Fluorophenyl sulfone, relative to B 3, tris (4-Fluorophenyl) phosphine oxide. The A 2 oligomers were synthesized by reaction of Bisphenol-A and B 2, in the presence of the B 3 unit, at temperatures between 100 and 160 °C, followed by an increase in the reaction temperature to 180 °C at which point the branching unit was incorporated. The presence of branching was confirmed via 31 P NMR spectroscopy and the thermal properties of the polymers were evaluated utitilizing TGA and DSC analyses. iii Table of Contents 1. Introduction…………………………………………………………………………….1 Linear polymers………………………………………………………...…2 Dendrimers………………………………………………………………...5 Branched poymers………………………………………………………...9 Poly(arylene ether sulfone)s……………………………………………..14 Current project…………………………………………………………...17 2. Experimental…………………………………………………………………………..19 Synthesis of 4,4-bis -(4-methylphenoxy)diphenyl sulfone, 1b ……………20 Synthesis of mono-substituted tris -(4-Fluorophenyl)phosphine oxide, 3a ………...………………………………………..20 Representative procedure for model reactions…………………………...20 Representative polymerization procedure………………………………..21 General procedure for reverse precipitations……………………….…....21 Preparation of GC/MS calibration curve………………………………...22 Polymerization of A 2 + B 2 +B 2’ monomers, linear analog………………22 Synthesis of A 2 capped oligomers, 2a…………………………………...22 Synthesis of branched poly(arylene ether sulfone)s, 6g …………………23 3. Results and Discussion………………………………………………………………..24 Nucleophilic aromatic substitution………………………………………24 Reactivity determination…………………………………………………24 GC/MS calibration curve………………………………………………...26 Simulation of in situ oligomeric A 2 formation…………………………..30 iv Synthesis of branched poly(arylene ether sulfone)s……………………..33 Incorporation of B 3 monomer……………………………………………38 Estimation of degree of branching, DB …………………………………..39 Thermal Analysis………………………………………………………...44 Conclusions………………………………………………………………47 4. References……………………………………………………………………………..48 v List of Figures I. Introduction: Figure 1 . Chain Entanglements……………………………………………………...…....1 Figure 2 . Intrinsic or inherent viscosity versus M n for a generic linear polymer……...…2 Figure 3 . Young’s Modulus versus temperature plot for a generic linear polymer…........3 Figure 4 . Poly(amido amine)……………………………..………………………….…...6 Figure 5 . Generic scheme of a branched polymer…………...…………………………...9 Figure 6 . Comparison of mechanical properties versus degree of branching for linear, dendritic and branched polymers………………………………………………………...10 Figure 7a . Comparison of linear, dendritic and branched polymer viscosity versus degree of branching……………………………………………………………………………...11 Figure 7b . Comparison of linear, dendritic and branched polymer viscosity versus Mw………………………………………………………………………………………..11 II. “One-Pot” Oligomeric A 2 + B 3 Polymerization of Poly(arylene ether sulfone)s: Reactivity Ratio Controlled Polycondensation Figure 8 . 4-Fluorophenyl sulfone, tris-(4-Fluorophenyl)phosphine oxide and bis-(4- Fluorophenyl)phenyl phosphine oxide…………………………………………………..25 1 Figure 9 . The 300 MHz H NMR spectrum (CDCl 3) of 1b ……………………………..27 13 Figure 10 . The 75 MHz C NMR (CDCl 3) spectrum of 1b …………………………….28 31 Figure 11 . The 121 MHz P NMR (CDCl 3) spectrum of 3a mixture…………………..30 Figure 12 . GPC overlay of 6b and 6b 1…………………………………………………..34 Figure 13 . Overlay of “one-pot” polymers, 6a-f………………………………………..35 Figure 14 . Overlay of MeOH soluble, insoluble and crude polymer……………………36 vi Figure 15 . Overlay of 6d , 6f and 6g ……………………………………………………..38 1 Figure 16 . The 300 MHz H NMR spectrum (CDCl 3) of 6c ……………………………39 Figure 17 . Potential B 3 structural units…………………………………………………40 31 Figure 18 . The 121 MHz P NMR (CDCl 3) spectrum of 6d mixture…………………..41 Figure 19 . Overlay of 31 P NMR spectra of 6a-h………………………………………...43 Figure20 Overlay of TGA traces under N 2………………………………...……………45 Figure 21 . Overlay of DSC spectra for samples 6a-h…………………………………...46 vii List of Schemes I. Introduction: Scheme 1 . Divergent and convergent methods for synthesis of dendrimers…………....8 Scheme 2 . Various syntheses of hyperbranched polymers...............................................13 Scheme 3 . Generic synthesis of polysulfones…………...………………………………15 Scheme 4. Generic synthesis of the oligomeric A 2 + B 3 system………………………...16 Scheme 5 . Synthesis of branched PAES via the oligomeric A 2 + B 3 system……………17 II. “One-Pot” Oligomeric A 2 + B 3 Polymerization of Poly(arylene ether sulfone)s: Reactivity Ratio Controlled Polycondensation Scheme 6 . NAS mechanism……………………………………………………………..34 Scheme 7 . Synthesis of 1b ……………………………………………………………….27 Scheme 8 . Synthesis of phosphoryl based compounds 3a-c…………………………….29 Scheme 9 . Model Reaction Scheme……………………………………………………..31 Scheme 10 . Conversion of 3 to 2a as an AB 2 oligomer………………………………...32 Scheme 11 . Branched PAEs polymer synthesis…………………………………………33 Scheme 12 . Synthesis of PAEs via “two-pot” literature method………………………..37 Scheme 13 . Synthesis of 6h ……………………………………………………………...37 viii List of Tables I. “One-Pot” Oligomeric A 2 + B 3 Polymerization of Poly(arylene ether sulfone)s: Reactivity Ratio Controlled Polycondensation Table 1. Reactivity data of 4-Fluorophenyl sulfone, tris(4-Fluorophenyl)phosphine oxide and bis-(4-Fluorophenyl)phenylphosphine oxide……………………………………….26 Table 2 . GC/MS data of model reactions………………………………………………..32 Table 3 . Reaction conditions utilized for the preparation of branched PAEs…………...33 Table 4 . Mn and PDI values of PAEs…………………………………………………...44 Table 5 . Incorporation of B 3 monomer………………………………………………….39 Table 6 . Degree of branching values…………………………………………………….42 Table 7 . TGA and DSC data for 6a-h…………………………………………………...45 ix Acknowledgements I would first like to thank Dr. Eric Fossum for his guidance, support and encouragement throughout my career at Wright State University as well as the faculty and staff of the Chemistry department. Also, many thanks to my husband, Nick, my parents, Jack and Donna, and to my sister, Laura. This could not have been accomplished without their endless love and support. x INTRODUCTION Linear Polymers Linear polymers are the most basic of polymers, consisting of only a simple back bone with two end groups. While linear polymers all have the same global structure, their individual structure will influence the conformation they exhibit. For example, poly (p- phenylene terephthalamide) will exhibit a rigid-rod like structure. 1 This means the polymer is in a defined rod-like shape and very little movement is allowed. On the other hand, polymers with a structure which has flexible bonds, as in poly(ether)s, will allow for random coil behavior, meaning there is no defined pattern within the structure of the polymer. While movement in rigid rod polymers is restricted, random coil polymers can participate in chain entanglements, where the chains become intertwined with one another or themselves ( Figure 1 ). Specifically, these chain entanglements bestow mechanical properties to the polymer. Figure 1 . Chain Entanglements. Intermolecular forces can also play a role in the ability of polymers to participate in chain entanglements. While the structure of the polymer may not be rigid on its own, 1 when enough time is allowed, the polymer chains can align their intermolecular forces to form a crystalline material. For example, poly(ethylene terephthalate) can either be amorphous or crystalline depending on the time allotted for cooling. If cooled slowly, crystallites will form and the polymer’s amorphous material will be “tied down” by the crystalline material until the crystallites melt. In this semi-crystalline material, only the small portions between crystallites can have chain entanglements, significantly reducing the total number of chain entanglements within the polymer and therefore also changing the mechanical properties. While many factors influence the ability of a polymer to participate in chain entanglements, the number-average molecular
Details
-
File Typepdf
-
Upload Time-
-
Content LanguagesEnglish
-
Upload UserAnonymous/Not logged-in
-
File Pages62 Page
-
File Size-