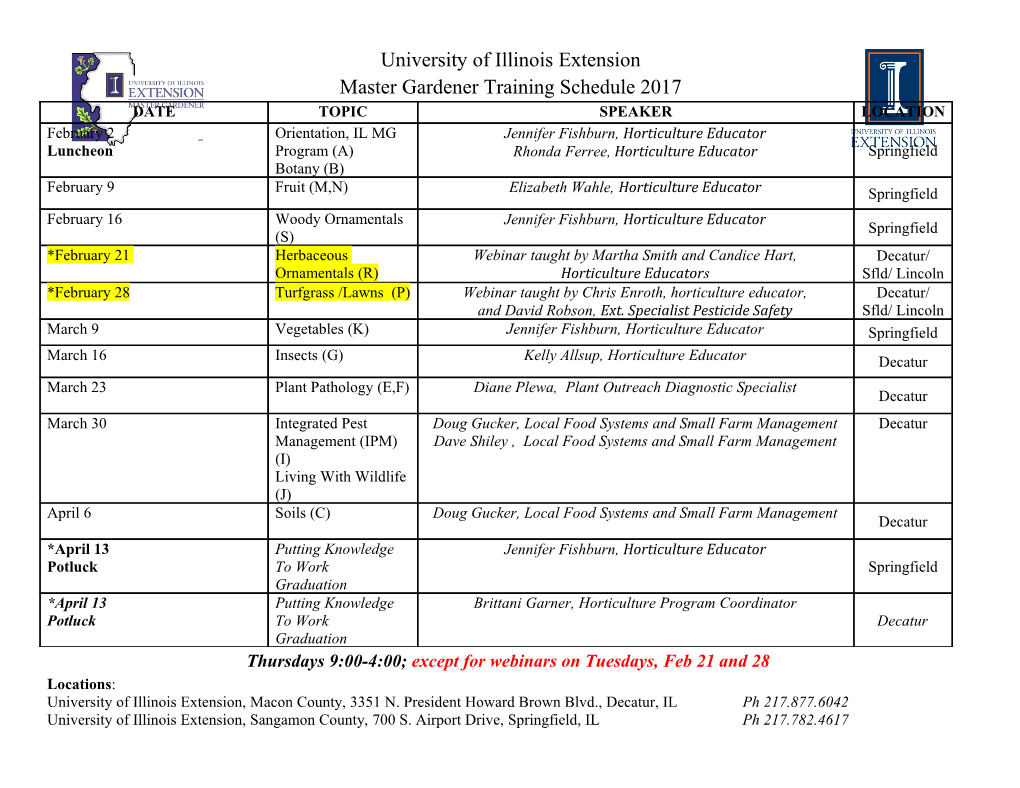
REVIEW Advanced Applications of MRI in Human Brain Science Verne S. Caviness, Jr.,1 Nikos Makris,1 Nicholas T. Lange,2 Martha Herbert1 and David N. Kennedy1,3 Departments of 1Neurology, Massachusetts General Hospital, 2Department of Psychiatry, McLean Hospital, and 3 Department of Radiology, Massachusetts General Hospital, Harvard Medical School, Boston, MA, USA (Received for publication on December 14, 1999) Abstract. Magnetic resonance imaging of the brain is now generally indispensable to state of art clinical medicine. Robust, high resolution imaging systems are currently available worldwide. The availability of MRI has, in little more than a decade, revolutionized the certainty and efficiency of clinical diagnosis and management. As a dividend of this revolution, clinicians and radiologists who are expert in the many and varied applications of MRI methods are able to relate this expertise to a con fident mastery of the topographic anatomy of the brain as revealed in magnetic resonance images. Whereas the yield to clinical objectives has been massive, the clinician as yet draws upon a relatively limited sampling of the potential informational harvest from this technology which in theory could further enrich both clinical concerns and those of fundamental neuroscience. Here we will review early explorations into these other offerings with the expectation that the coming decade will see them established comfortably with current uses. We will also consider potential offerings of the extended applications of brain MRI to the characterization and insights into biological origins of certain obscure developmental disorders. (Keio J Med 49 (2): 66-73, June 2000) Key words: MRI, brain morphometry, neural systems, developmental disorders Three Stages of Application shape of the principal cerebral, brain stem and cere bellar regions and the gray scale compartments of MR represents the brain as a range of gray scale these regions match or do not match those of the images which may be formatted in any or all three of standard of normal brain. This level of application is the cardinal coronal, axial or sagittal planes. Planes may ufficient to those requirements needed to judge many be sampled only selectively from the brain with variable developmental malformations as abnormal.1 It is suffi plane thickness and interplane gaps or the image set cient for the recognition of stroke or tumor. may be fully 3 dimensional. The imaged set is an alge braic transform of the imaged brain. Respecting these Knowledge-based electively obtained properties of an image data set, we consider three potential stages of analytic operation. Here the observer, drawing upon a base of knowl These stages build, one upon the other, a systematic edge of the structure, organization and development of interpretation and view of the human brain as imaged the normal brain, looks beyond the raw gray scale and by the MRI system. shape presentation of the brain and makes inferences in these domains from the image presentation of pattern Pattern recognition (Fig. 1b). The interpretations of image pattern provided by the skilled radiologist and clinician will be richly By pattern recognition we intend judgements made reinforced by such knowledge. Conceptual frameworks practically with reference only to the gray-white pat and technologies only now beginning to mature will terns as viewed in the images (Fig. 1a). That is, the soon contribute much more at this level of analysis. judgement is made as to whether the gray scale and The critical issue here is the relationship of neural Presented at the 1140th Meeting of The Keio Medical Society in Tokyo, November 9, 1999. Reprint requests to: Dr. V. S. Caviness, Jr., Department of Neurology, Massachusetts General Hospital, Harvard Medical School, Boston , MA 02114, USA, e-mail: [email protected] 66 Keio J Med 2000; 49 (2): 66-73 67 Fig. 1 MRI image of the brain. a) A coronal Tl-weighted MRI image at the level of the head of the caudate. b) Results of anatomic segmentation of this image are demonstrated. Unique anatomic regions are color coded and labeled. Abbreviations: Fl, first frontal gyrus; F2, second frontal gyrus; Cga, anterior cingulate gyrus; PAC, paracingulate gyrus; PRG, precentral gyrus; TP, temporal pole; INS, insula; CO, central operculum; FOC, frontoorbital cortex; Aput, anterior putamen; CauH, head of caudate; NA, nuculeus accumbens; V, ventricle. structure and of neural systems organization to what is gyri, the nuclear masses according to approximate actually visible in the gray scale MR images. For ex nuclear groups and the white matter into strata which ample the continuous cortical gray compartment at the distinguish the principal fascicular groupings.9-12 For surface of the cerebral hemisphere includes neocortex, each gray and white matter parcellation unit, generally archicortex and paleocortex.2 The extent of each of each only a few percent or less of the total volume of these structures is revealed by reliable topographic the hemisphere, we have formulated an atlas of systems landmarks, principally with reference to hemispheric related structural components. For each gray matter location and the local course and intersections of fis parcellation unit, whether cortical or subcortical, we sures. The neocortex is thrown into convolutions which have developed a reasoned atlas of principal con in the normal brain conform to a canonical pattern, nectivity, based upon dissection and, by extrapolation, though with substantial variations in the details of this upon hodologic experiment in primates. For each par pattern among individuals.3 The neocortex internally is cellation unit of white matter there is a companion atlas composed of several dozen architectonic fields, each of traversing principal fascicles given both in terms of with its specific role in the systems operation of the provenance and destination (Fig. 2). Standard anatomic forebrain. To a substantial extent there is good corre images may be complemented to this end by explicit 3 lation between specific gyrus and the location of archi dimensional demonstration of specific fiber tracts by tectonic field.4-6 Central gray masses, similarly, are di diffusion tensor imaging (Fig. 3).13 Finally, this presen visible into nuclei by local topographic landmarks.7 tation may be complemented by spectroscopy, EEG or Finally, the white matter is subcompartmentalized re MEG where the overlays from these additional tech specting the course and quality of principal associative, nologies may be registered computationally with those projection and commissural axon fascicles, and the of the basic 3 dimensional anatomic image sets (Fig . course of specific fascicles is systematically and reliably 4)14.15 positioned with respect to the three dimensional con Applications in general will apply to any analysis formation of the white matter stratification.8 where the objective is to deduce inferences about sys The extensive knowledge base which correlates top tems organization from the pattern data set. In the case ographic anatomy of the human brain with gray scale of lesion analysis in cognitive neuroscience , for exam MR images may be tapped only after extensive proc ple, the method first characterizes the lesion itself in essing of the topographic anatomy. We illustrate here in terms of the underlying gray and white matter struc outline an approach that has usefully allowed such cor tures destroyed where characterization extends to the relations. In brief, we work from a computer assisted domains of localization and size.16,17 In the domain of system of analysis that allows us first of all to segment connectivity, an estimate of interrupted connectivity apart, the gray, white and CSF compartments and to may be inferred from the mapping of destroyed gray partition the neocortex according to the principal set of and white matter parcellation units with reference to 68 Caviness VS, et al: MRI and the Human Brain Fig. 2 Maps of anatomic connectivity. a) The cortical connections of the commissural systems, including the corpus callosum, anterior commissure (ac) and dorsal hippocampal commissure (dhc) are shown. The color coding of the cortical regions corresponds to the color coding of the commissural regions. b) This figure shows the map of cortical anatomic connectivity (MAC) for the cingulum bundle (CB). Abbrevia tions: AG: angular gyrus; CALC: intracalcarine cortex; CGa: cingulate gyrus, anterior; CGp: cingulate gyrus, posterior; CN: cuneal cortex; CO: central operculum; F1: superior frontal gyrus; F2: middle frontal gyrus; F3o: inferior frontal gyrus, pars opercularis; F3t: inferior frontal gyrus, pars triangularis; FMC: frontal medial cortex; FO: frontal operculum; FOC: frontal orbital cortex; FP: frontal pole; Hl: Heschl's gyrus; INS: insula; JPL: juxtaparacentral cortex; LG: lingual gyrus; OP: occipital pole; OF: occipital fusi form gyrus; OLi: lateral occipital cortex, inferior; OLs: lateral occipital cortex, superior; PAC: para cingulate cortex; PCN: precuneus; PHa: parahippocampal gyrus, anterior; PHp: parahippocampal gyrus, posterior; PO: parietal operculum; POG: postcentral gyrus; PP: planum polare; PRG: precentral gyrus; PT: planum temporale; SC: subcallosal cortex; SCLC: supracalcarine cortex; SGa: supramarginal gyrus, ante rior; SGp: supramarginal gyrus, posterior; SPL: superior parietal lobule; T1a: superior temporal gyrus, anterior; Tip: superior temporal gyrus, posterior; T2a: middle temporal gyrus, anterior; T2p: middle temporal gyrus, posterior;
Details
-
File Typepdf
-
Upload Time-
-
Content LanguagesEnglish
-
Upload UserAnonymous/Not logged-in
-
File Pages8 Page
-
File Size-