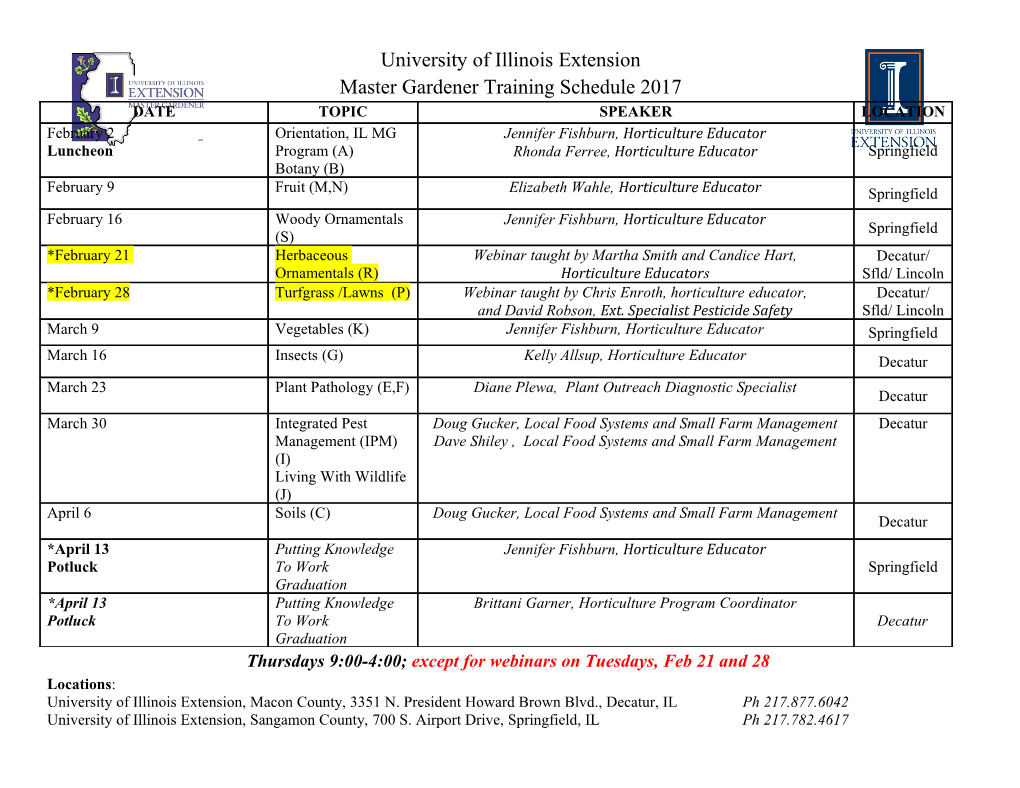
Department of Physics PhD School in Physics, Astrophysics and Applied Physics Cycle XXXIII Constraints on neutron star structure from pulsar glitches Disciplinary Scientific Sector FIS/05 Supervisor: Prof. Pierre M. Pizzochero Co-supervisor: Dr. Marco Antonelli School Director: Prof. Matteo Paris PhD Thesis of: Alessandro Montoli Academic year 2020–2021 Final examination committee: External Referee: Doctor Cristóbal M. Espinoza, Universidad de Santiago de Chile, Departamento de Física External Referee: Professor Andrew Melatos, University of Melbourne, School of Physics External Member: Professor Francesca Gulminelli, Université Caen Normandie, LPC/ENSICAEN, CNRS/IN2P3 External Member: Professor Nils Andersson, University of Southampton, Mathematical Sciences and STAG Research Centre External Member: Professor Michał Bejger, Nicolaus Copernicus Astronomical Center, Polish Academy of Sciences Final examination: Friday, December 18, 2020 Università degli Studi di Milano, Dipartimento di Fisica, Milano, Italy Cover illustration: Chart on which Jocelyn Bell Burnell first recognised evidence of PSR B1919+21, courtesy of the Churchill Archives Centre MIUR subjects: FIS/05 - Astronomia e Astrofisica PACS: 97.60.Gb Pulsars 97.60.Jd Neutron stars 26.60.-c Nuclear matter aspects of neutron stars Keywords: stars: neutron, pulsars: general, dense matter, gravitation, pulsars: indi- vidual: PSR J0835-4510, superfluidity, glitch, Vela Pulsar, neutron stars masses Abstract Neutron stars are among the densest objects in the Universe, making them a perfect laboratory to study nuclear matter under extreme conditions. Pulsars – rapidly rotating magnetised neutron stars – are one of their possible manifestations, being observed as an extremely regular periodic emission in the radio spectrum. This radiation is produced by converting their rotational energy and, because of this, pulsars are expected to spin down. Some of them, however, have been observed exhibiting sudden accelerations in their rotation, also known as glitches. Nowadays, pulsar glitches are interpreted as the manifestation of vortex dynamics in the internal neutron superfluid, which lags behind the observable charged component in spinning down, occasionally releasing angular momentum to it and giving rise to a glitch. In this work, we will present three different observational characteristics of a glitching pulsar – its largest glitch, its average acceleration due to glitches and its short-time evolution after a glitch – and we will try to extract information about the neutron star from each of them. In particular, we will try to set constraints on the mass of the star, the moment of inertia of its reservoir component and several other quantities tied to the glitch phenomenon, with the ultimate goal of increasing our knowledge about the properties of matter at densities above those of terrestrial nuclei. i Contents Introduction1 1 Neutron stars overview5 1.1 Brief history of neutron stars5 1.2 From main sequence to compact objects8 1.3 Structure 10 1.4 Equations of state 12 1.5 Neutron star phenomenology 17 1.6 Pulsar timing and pulsar glitches 22 2 Largest observed glitch 35 2.1 Analytical calculation 35 2.2 Numerical results 43 3 Activity parameter 53 3.1 Calculation of the activity parameter 53 3.2 Moment of inertia constraint 64 3.3 Revised maximum glitch mass constraint 69 4 Angular velocity evolution 93 4.1 Three-component model 94 4.2 Application to the 2016 Vela glitch: frequentist approach 104 4.3 Application to the 2016 Vela glitch: Bayesian approach 112 Future directions 125 iii iv Contents Appendices 129 A Moments of inertia in the slow rotation approximation 131 Bibliography 141 List of Publications 159 Introduction Since the first speculations about their existence in the Thirties and the first theoretical studies done until their discovery in the late Sixties, many advances have been made in the description of neutron stars, one of the possible remnants of an ordinary star, and more specifically its core after a core-collapse supernova. With a mass of approximately 1.5M , packed by gravity in a sphere of radius 10 km, neutron stars are among the densest objects in the Universe and can easily exceed≈ the nuclear saturation density, i.e. the density inside heavy atomic nuclei. In fact, it is not infrequent to come across models which predict central densities ten times larger than this value and claim the existence of exotic matter, like hyperons, meson condensates and quark-gluon plasma. As it is not possible to achieve such densities on Earth, these objects are arguably one of the few ways to study nuclear matter above the saturation density, making them the perfect playground for nuclear physics. One of the most important consequences of these extreme densities is the onset of nuclear superfluidity, which causes many phenomena observable at macroscopic scale, such as pulsar glitches. Moreover, due to their extreme compactness, neutron stars experience extreme gravitational forces, so a complete and thorough description should be carried out in a General Relativistic framework. Their Schwarzschild radius is, in fact, approxi- mately 0.2 0.4 times their radius, meaning that spacetime is curved around and inside neutron stars− and their physical description is affected by important general relativistic corrections. Finally, neutron stars present an extremely varied phenomenology. Their emission spans the entire electromagnetic spectrum, ranging from radio frequencies to gamma rays, with both continuous and discontinuous emissions, both regular and sporadic. We can indirectly observe neutrino emission during the first stage of their lives, by measuring the cooling of their surface temperature over the years (see, e.g., the neutron star in the Cassiopeia supernova remnant, Heinke and Ho, 2010; Yakovlev et al., 2011), and directly detect it during the supernova phase (so far only in SN 1987A). They are also good candidates for emitting continuous gravitational waves, and it has also been possible to detect gravitational waves from neutron stars in binary systems. All these three lines of research about neutron stars – the nuclear one, the 1 2 Introduction one related to General Relativity and the observational one – are highly intertwined. In fact, it is not possible to obtain serious theoretical constraints or observational predictions on a neutron star phenomenon without keeping into account all these three aspects. As an example, the observation of a neutron star of about two solar masses (Demorest et al., 2010; Antoniadis et al., 2013; Fonseca et al., 2016; Linares et al., 2018; Cromartie et al., 2020) has put a serious constraint on the physics of nuclear matter over saturation density, and ruled out several models. One of the possible manifestations of neutron stars are pulsars, which are rapidly rotating neutron stars emitting a collimated beam of radiation. When this beam is pointed in the direction of Earth, we observe a pulse in the radio spectrum, much like a lighthouse. On average, this pulsation is extremely stable, even more stable than atomic clocks (Milner et al., 2019). These objects slow down, due to a hydromagnetic torque exerted by the pulsar wind they emit, except for sudden accelerations of their pulsation frequency. These phenomena are called glitches. The current interpretation of glitches is related to the presence of a neutron superfluid in the star interior, which lags behind the observable charged component in spinning down, effectively storing angular momentum. This angular momentum reservoir is occasionally released, giving rise to a glitch. It is clear that an accurate modelling of the glitch phenomenon is necessary in order to explain the observed glitch behaviour. On the other hand, the latter can be an important test for the input variables of the model. In other words, it is possible to constrain some of the characteristics of a neutron star with its glitching behaviour. This is the leitmotif of this thesis: each Chapter presents a different observational feature of pulsar glitches which can be employed to provide new information about the internal structure of neutron stars. With this in mind, we divide this thesis into four Chapters: Chapter1 We present here some key features of neutron stars, talking about structure, Equations of State, phenomenology and the basic modelling of pulsar glitches. Chapter2 Glitch modelling can be employed to find the maximum angular momen- tum reservoir that can be stored in a neutron star between glitches and, as a consequence, the largest glitch achievable by a pulsar. This value can be easily compared with the largest observed glitch of a particular object, and some con- straints can be set on the microphysical inputs of the model and on the mass of the star. All the modelling have been performed in the general relativistic framework. This Chapter is based on the work published in Antonelli et al. (2018). Chapter3 Pulsar activity is a well-known and widely used parameter in the field of pulsar glitches. It summarises the average acceleration of a neutron star’s rotation due to the presence of glitches. After some considerations about the calculation of this parameter, we present two different models. The first one (studied in a general relativistic framework and already well-known in the literature, see Link et al. 1999) constrains the moment of inertia of the superfluid component, and Introduction 3 the mass of a star as a consequence. The second one is an extension of the model presented in the preceding
Details
-
File Typepdf
-
Upload Time-
-
Content LanguagesEnglish
-
Upload UserAnonymous/Not logged-in
-
File Pages169 Page
-
File Size-