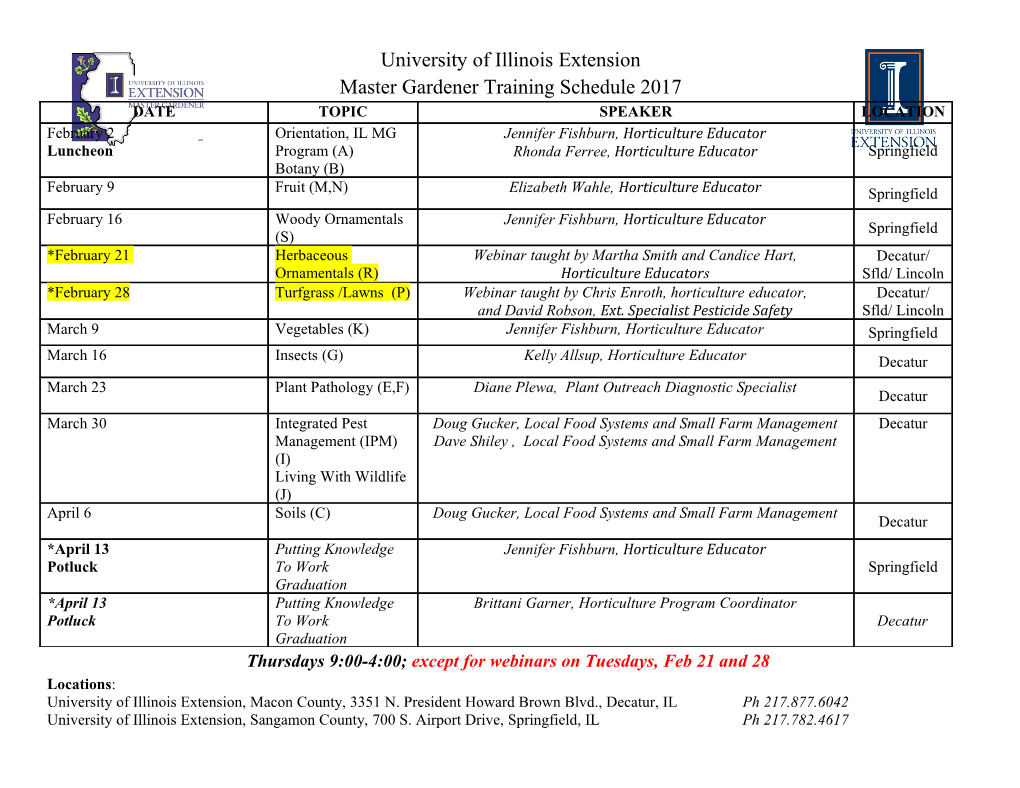
Proc. Natl. Acad. Sci. USA Vol. 95, pp. 3437–3442, March 1998 Biochemistry The crystal structure of a 3D domain-swapped dimer of RNase A at a 2.1-Å resolution (protein structureyx-ray crystallographyyprotein–protein interactionsyamyloid) YANSHUN LIU*, P. JOHN HART*, MICHAEL P. SCHLUNEGGER†, AND DAVID EISENBERG*‡ *University of California-Department of Energy Laboratory of Structural Biology and Molecular Medicine, Departments of Chemistry and Biochemistry and Biological Chemistry, University of California, Los Angeles, CA 90095-1570; and †Merck Sharp & Dohme-Chibret AG, Schaffhauserstrasse 136, CH-8152 Glattbrugg, Switzerland Contributed by David Eisenberg, December 31, 1997 ABSTRACT The dimer of bovine pancreatic ribonuclease subunit and vice versa. The term 3D domain swapping was A (RNase A) discovered by Crestfield, Stein, and Moore in coined to describe a dimer of diphtheria toxin (8). Since then, 1962 has been crystallized and its structure determined and more than 10 crystal structures of 3D domain-swapped pro- refined to a 2.1-Å resolution. The dimer is 3D domain- teins have been reported (9), including a trimer (10). The swapped. The N-terminal helix (residues 1–15) of each subunit interface between domains found both in the monomer and in is swapped into the major domain (residues 23–124) of the the domain-swapped oligomer is termed the ‘‘closed inter- other subunit. The dimer of bull seminal ribonuclease (BS- face,’’ and the interface found only in the oligomer is termed RNase) is also known to be domain-swapped, but the rela- the ‘‘open interface’’ (9, 11). These two types of interfaces are tionship of the subunits within the two dimers is strikingly illustrated in Fig. 2 later in this paper. 3D domain swapping can different. In the RNase A dimer, the 3-stranded beta sheets of endow oligomers with additional properties, e.g., metal bind- the two subunits are hydrogen-bonded at their edges to form ing in the case of CksHs2, a cell cycle regulatory protein in a continuous 6-stranded sheet across the dimer interface; in yeast (12). In addition, domain swapping is a possible mech- the BS-RNase dimer, it is instead the two helices that abut. anism for the formation of protein aggregates, which can be Whereas the BS-RNase dimer has 2-fold molecular symmetry, favored by mutational and environmental changes (9, 11). The the two subunits of the RNase A dimer are related by a rotation amyloid-like fibrils formed by intact Ig light chains have been ; of 160°. Taken together, these structures show that inter- suggested by Klafki et al. (13) to form by a mechanism that is subunit adhesion comes mainly from the swapped helical essentially domain swapping. Studies of oligomers of the well domain binding to the other subunit in the ‘‘closed interface’’ characterized protein RNase A may therefore illuminate the but that the overall architecture of the domain-swapped formation of amyloid and other protein aggregates. oligomer depends on the interactions in the second type of Bull seminal (BS)-RNase is a 124-residue ribonuclease from interface, the ‘‘open interface.’’ The RNase A dimer crystals bovine seminal plasma that shares 81% sequence identity with take up the dye Congo Red, but the structure of a Congo RNase A. The structure of BS-RNase shows it is a domain- Red-stained crystal reveals no bound dye molecule. Dimer swapped dimer (14). Domain swapping endows this dimer with formation is inhibited by excess amounts of the swapped additional biological properties, such as allostery (15), and helical domain. The possible implications for amyloid forma- antitumor and immunorepression activity (16, 17), functions tion are discussed. not possessed by the monomer and the non-domain-swapped dimer of BS-RNase. The mechanism for these acquired bio- Bovine pancreatic ribonuclease A (RNase A) is a 124-residue logical properties remains under study (18). Solution and enzyme that catalyzes the hydrolysis of RNA with specificity computational studies of BS-RNase and RNase A suggest that 9 for pyrimidines at the 3 -side of the cleavage site. Studies of the hinge loop (residues 16–22) connecting the swapped RNase A have made great contributions to our understanding domain (residues 1–15) and the major domain (residues 23– of protein structure and function, including early studies of the 124) permits the domain exchange, perhaps with Pro-19 in spontaneous renaturation of proteins (1), amino acid sequence BS-RNase being important (19–21). The structure of the determination (2), synthesis of proteins (3), protein three- RNase A monomer was first determined to2ÅbyKartha et dimensional structure (4, 5), and peptide–protein interactions al. in 1967 (4) and later refined to 1.26 Å by Wlodawer et al. (6). Here, we pursue another aspect of RNase A: its formation (22). Also, the structure of RNase S, the RNase A cleaved by of oligomers by 3D domain swapping. subtilisin, was determined by Wyckoff et al. (5). The structure In 1962, Crestfield et al. (7) reported that RNase A forms of the RNase A dimer, however, has gone undetermined until dimers and higher oligomers after dialysis against 50% acetic now. acid and lyophilization. The dimer remains active with the Our rationale for studying binding of Congo Red to the same specific activity as the monomer. From studies of chem- RNase A dimer is the possible relationship of domain swap- ical modification of active site residues, Crestfield et al. pro- ping to amyloid formation, mentioned above. Congo Red is a posed that RNase A forms a dimer by exchanging its N- clinical probe for amyloid; when it binds to amyloid a green terminal helix (7), a phenomenon now called 3D domain swapping (8). In a 3D domain-swapped dimer, two subunits exchange Abbreviations: RNase A, bovine pancreatic ribonuclease A; BS- RNase, bull seminal ribonuclease. identical ‘‘domains.’’ That is, a structural unit of one subunit Data deposition: The atomic coordinates and structure factors have takes the place of the identical structural unit of the other been deposited in the Protein Data Bank, Biology Department, Brookhaven National Laboratory, Upton, NY 11973 (reference The publication costs of this article were defrayed in part by page charge 1A2W). ‡To whom reprint requests should be addressed at: UCLA-DOE payment. This article must therefore be hereby marked ‘‘advertisement’’ in Laboratory of Structural Biology and Molecular Medicine, Depart- accordance with 18 U.S.C. §1734 solely to indicate this fact. ments of Chemistry and Biochemistry and Biological Chemistry, © 1998 by The National Academy of Sciences 0027-8424y98y953437-6$2.00y0 University of California, Los Angeles, Box 951570, Los Angeles, CA PNAS is available online at http:yywww.pnas.org. 90095-1570. e-mail: [email protected]. 3437 Downloaded by guest on September 27, 2021 3438 Biochemistry: Liu et al. Proc. Natl. Acad. Sci. USA 95 (1998) birefringence is given off under polarized light (23). Several Initially, cryogenic x-ray diffraction data to a 2.8-Å resolu- models of Congo Red binding to amyloid have been proposed tion were collected with a Rigaku R-AXIS IV imaging plate (24, 25), but further structural evidence is needed to under- detector. Data to a 2.1-Å resolution were collected with an stand this interaction. We observed that Congo Red stains R-AXIS II imaging plate detector at room temperature with a crystals of the RNase A dimer, which led us to examine the larger crystal (0.1 3 0.1 3 0.5 mm3). The x-ray source was a crystal structure of a potential RNase A–Congo Red complex. Rigaku RU-200 generator running at 50 kV, 100 mA with There exists a subtilisin cleavage site between residues 20 focusing mirrors. Crystals were rotated about f, and oscilla- and 21 in RNase A. After cleavage, the N-terminal segment, tion images were collected every 1.5°. The data were processed the S-peptide, remains associated with the rest of the protein, and reduced using the programs DENZO and SCALEPACK (26). the S-protein (6). Low pH is required to separate the S-peptide The statistics of data collection are listed in Table 1. from the S-protein. Because the swapped helices in the RNase Structure Determination. The structure of the RNase A A dimer are contained within the S-peptide, exogenous S- dimer was determined by molecular replacement method using peptide may compete with the formation of the dimer. Studies AMORE (27). The search model was the RNase A monomer of this competition may thus illuminate the mechanism of (28) without the hinge loop. The BS-RNase dimer (14) failed as a search model, for reasons which the structure now makes domain swapping. s Our structural study of the RNase A dimer was undertaken clear. Model building was undertaken using all data (no to see if it is domain-swapped, and if so, to compare its mode cutoff) during 20 rounds of crystallographic refinement in of 3D domain swapping with the BS-RNase dimer. Other goals XPLOR (29). A ‘‘round’’ of refinement is defined as sequential included studying the mechanism of binding of Congo Red to application of positional, simulated annealing and isotropic amyloids and the formation of protein aggregates. temperature factor (B factor) refinement, followed by visual inspection of electron density maps coupled with manual model building using the molecular graphics program FRODO METHODS (30). The solvent molecules were added using program XTAL Purification of the RNase A Dimer. The RNase A dimer was VIEW (version 4.0) (31). Model atom positions of the hinge formed and isolated as reported by Crestfield et al. (7). RNase loops were verified by the examination of conventional and simulated annealing omit maps (32). A was purchased from Sigma (RNase A III-A).
Details
-
File Typepdf
-
Upload Time-
-
Content LanguagesEnglish
-
Upload UserAnonymous/Not logged-in
-
File Pages6 Page
-
File Size-