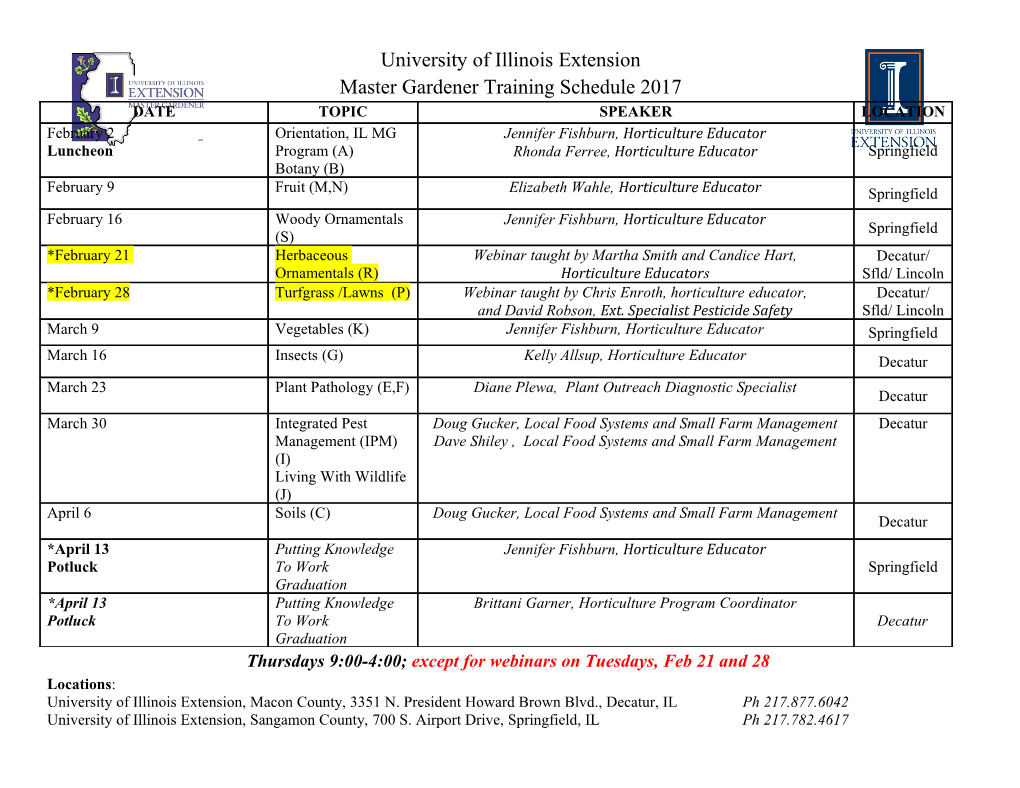
DEGREE PROJECT IN VEHICLE ENGINEERING, SECOND CYCLE, 30 CREDITS STOCKHOLM, SWEDEN 2018 Preliminary Lander CubeSat Design for Small Asteroid Detumbling Mission AGNE PASKEVICIUTE KTH ROYAL INSTITUTE OF TECHNOLOGY SCHOOL OF ENGINEERING SCIENCES Preliminary Lander CubeSat Design for Small Asteroid Detumbling Mission AgnėPaökevičiūtė Department of Aeronautical and Vehicle Engineering KTH Royal Institute of Technology This thesis is submitted for the degree of Master of Science October 2018 Skiriu ö˛ darbπsavo mamai Graûinai ir tėčiui Algirdui. Acknowledgements This thesis would have not been possible without the encouragement and support of numerous people in my life. I would especially like to thank Dr Michael C. F. Bazzocchi for his support and constructive advice throughout my research. In addition, I would like to express my gratitude to researchers, professors and staffat Luleå University of Technology, Space Campus, for their ideas and help in practical matters. I would like to sincerely thank my family, boyfriend, and friends for believing in me, encour- aging me to reach for my dreams, and loving me without any expectations. Last but not least, I am truly grateful for the opportunities KTH Royal Institute of Technol- ogy provided. Sammanfattning Gruvdrift på asteroider förväntas att bli verklighet inom en snar framtid. Det första steget är att omdirigera en asteroid till en stabil omloppsbana runt jorden så att gruvteknik kan demon- streras. Bromsning av asteroidens tumlande är en av de viktigaste stegen i ett rymduppdrag där en asteroid ska omdirigeras. I detta examensarbete föreslås en preliminär asteroidlandare baserad på CubeSat-teknik för ett rymduppdrag där en asteroid ska omdirigeras. En asteroid av Arjuna-typ, 2014 UR, med en diameter på mellan 10.6 och 21.2 m är vald som kandidat för rymduppdraget. På grund av att asteroidens är relativt liten till storlek måste landningen utföras med en aktiv reglermetod och rymdfarkosten måste förankras till asteroiden. Med hjälp av en beslutsmetod utifrån flera mål, PROMETHEE, identifierades förankringsme- toden “mikro-ryggrads-gripare” som den mest lämpliga. Tre huvuduppgifter för rymduppdraget identifierades under designprocessen: dataflöde mellan landaren och moderfarkosten, Delta-V-budgeten och peknoggrannheten. Delta-V som krävs för landning på asteroiden uppskattas att vara högst 10 m/s. Bromsningen av tumlandet kostar högst 15 m/s. Osäkerheten med Delta-V för bromning av tumlandet beror på olika up- pskattningar av asteroidens storlek. Den nödvändiga minsta peknoggrannheten uppskattades vara 6°. Utformningen av landaren, baserade på CubeSat-teknik, använder till största delen kom- ponenter som finns på hyllan, s.k. commercial-off-the-shelf. Det visas att en CubeSat-landare inte kan bromsa tumlandet för en asteroid som roterar snabbt kring flera axlar. Om den valda asteroiden roterar runt en axel med en rotationsperiod på 2.4 timmar, är det möjligt att bromsa tumlandet med endast 1.5 kg drivmedel. Den föreslagna landaren är en 12U CubeSat med en total massa på 15 kg och strömförbrukning på 65 W. Abstract Asteroid mining is expected to become reality in the near future. The first step is to redirect an asteroid to a stable Earth orbit so that mining technologies can be demonstrated. Detumbling of the asteroid is one of the important steps in asteroid redirection missions. In this thesis, a preliminary lander CubeSat design is suggested for a small asteroid detumbling mission. The candidate asteroid for the detumbling mission is chosen to be 2014 UR, an Arjuna- type asteroid with an estimated diameter ranging from 10.6 to 21.2 m. Due to the small size of the asteroid, the landing must be performed with an active control method after which the spacecraft must be firmly anchored to the asteroid. By using the multi-criteria decision mak- ing method PROMETHEE, the microspine gripper is chosen as the most suitable anchoring mechanism. Three main mission drivers are identified during the design process: data-flow between the lander and the mothership, Delta-V budget and pointing accuracy. The Delta-V required for landing on the asteroid and despinning it is estimated to be 10 m/s and 0.15 m/s at most, respectively. The uncertainty with the despinning Delta-V is due to varying estimates of the size of the asteroid. The required minimum pointing accuracy is estimated to be 6◦. The preliminary lander CubeSat design can be largely realised with commercial off-the- shelf components suggested in this work. Only some of the components have to be custom built or the technologies further developed. It is shown that a CubeSat lander is not able to detumble an asteroid that is rotating fast around multiple axis. However, if the considered asteroid is rotating around a single axis with a rotational period of 2.4 h, it is be possible to despin it by spending just 1.5 kg of propellant. The suggested lander is a 12U CubeSat with an overall mass of 15 kg and power consumption of 65 W. Contents List of Figures ix List of Tables xi Nomenclature xiii 1 Introduction 1 1.1 Literature Review . 1 1.2 Motivation . 3 1.3 Preliminary Mission Description . 4 1.4 Thesis Overview . 5 2 Target Asteroid 6 2.1 Spectral Types of Asteroids . 6 2.2 Near-Earth Asteroids . 7 2.3 Asteroid 2014 UR . 10 2.3.1 Spectral Type of 2014 UR . 11 2.3.2 Surface Properties . 12 3 Forces Acting on The Landed Spacecraft 13 3.1 Introduction . 13 3.2 Gravitational Force . 16 3.3 Solar Radiation Pressure . 16 3.4 Electrostatic Force . 16 3.5 Centrifugal Force . 17 3.6 Despinning Force . 19 3.7 Net Force Acting on The Spacecraft . 21 Contents vii 4 Landing Systems Review and Choice 23 4.1 Literature Review . 23 4.1.1 Active Descent . 24 4.1.2 Passive Descent . 26 4.2 Landing Mechanism Selection . 27 5 Anchoring Systems Review 29 5.1 Literature Review . 29 5.1.1 Slow Anchoring Methods . 30 5.1.2 High-Speed Anchoring Methods . 32 5.2 Criteria for Successful Anchoring . 37 5.3 Discussion of Suitable Anchoring Systems . 37 5.3.1 Summary of Anchoring Systems Candidates . 38 6 Anchoring System Choice Using MCDM 41 6.1 Introduction . 41 6.2 Criteria Description . 43 6.3 Criteria Weights . 46 6.3.1 Optimal Pairwise Comparison . 47 6.3.2 Weights of Criteria . 50 6.4 Method of Aggregation . 51 6.4.1 PROMETHEE Method . 52 6.4.2 Discussion . 56 7 Primary Mission Drivers 57 7.1 Mission Data-Flow . 57 7.2 Delta-V Budget . 59 7.2.1 Sphere of Influence . 59 7.2.2 Delta-V Estimation: Docking . 60 7.2.3 Delta-V Estimation: Despinning . 62 7.3 Pointing accuracy . 62 8 Preliminary Spacecraft Design 64 8.1 Attitude and Orbit Control Subsystem . 64 8.1.1 Actuators . 65 8.1.2 Sensors . 67 8.2 Propulsion Subsystem . 69 Contents viii 8.2.1 Propellant Budget . 69 8.2.2 Subsystem Choice . 71 8.3 Anchoring Subsystem . 74 8.4 Communications Subsystem . 75 8.5 Other Subsystems . 76 8.5.1 Command and Data Handling Subsystem . 76 8.5.2 Thermal Subsystem . 77 8.5.3 Structure . 77 8.6 Power Subsystem . 77 8.7 Mass and Power Budget . 79 9 Conclusion 81 Bibliography 84 Appendix A Technology Readiness Levels 91 Appendix B Multi-Criteria Decision Making 92 B.1 Pairwise Comparison of Anchoring Criteria . 92 B.2 MATLAB Script for Pairwise Difference Comparison . 97 B.3 PROMETHEE Preference Functions and Parameters . 100 Appendix C Clohessy-Wiltshire Equations 103 C.1 Coordinate System . 103 C.2 Equations of Motion . 104 Appendix D Preliminary Design & Subsystems 106 D.1 Moment Arm & Moment of Inertia . 106 List of Figures 1.1 Operational concept sketch. Adapted from Probst and Förstner [1]. 5 2.1 Three subgroups of NEAs and their orbits with respect to Earth’s orbit around the Sun [2]. ................................... 8 3.1 Schematic diagram showing the lander on the surface of asteroid with the main natural forces acting on it. 14 3.2 Resultant centrifugal force distribution on asteroid 2014 UR for the worst case scenario. 18 3.3 Best case scenario force and time required for despinning the asteroid. 19 3.4 Landing location and direction of forces required for despinning the asteroid in the worst case scenario. 20 3.5 Worst case scenario force and time required for despinning the asteroid. 21 4.1 GRASP lander developed by SFL [3]. ..................... 24 4.2 Spinning lander concepts suggested by SCSG. 25 4.3 Surface mobility mechanisms patented by University of California [4]. 26 4.4 MASCOT mobility concept - eccentric arm concept [5]. 27 5.1 Sample self-opposing drill systems [6]..................... 31 5.2 Experimental setup of robotic arm presenting anchoring technology based on sawing method [7]. ............................... 32 5.3 Left: NASA JPL microspine grippers being tested for anchoring strength at 45◦. Right: CAD view of microspine gripper cross-section [8, 9]. 33 5.4 Telescoping spike anchoring system [10]. ................... 34 5.5 Telescoping spike system for ST4/Champollion mission [10]. 35 5.6 Tethered spike anchoring system [10]. ..................... 36 5.7 Philae harpoon anchoring system mounted in the landing gear [11]. 36 5.8 Magnetic anchoring concept “Harvestor” by Deep Space Industries [6]. 37 List of Figures x 6.1 Flowchart for the MCDM methodology. 42 7.1 Data-flow diagram for asteroid detumbling mission. Round shapes denote data source, rectangles denote tasks, hexagon denotes data end user. 58 7.2 Delta-V required to dock with asteroid 2014 UR versus rendezvous time. 61 7.3 Scheme for estimating required maximum pointing angle to the asteroid 2014 UR, just after spacecraft release from the mothership. 63 8.1 Attitude control motion in two dimensions [12].
Details
-
File Typepdf
-
Upload Time-
-
Content LanguagesEnglish
-
Upload UserAnonymous/Not logged-in
-
File Pages126 Page
-
File Size-