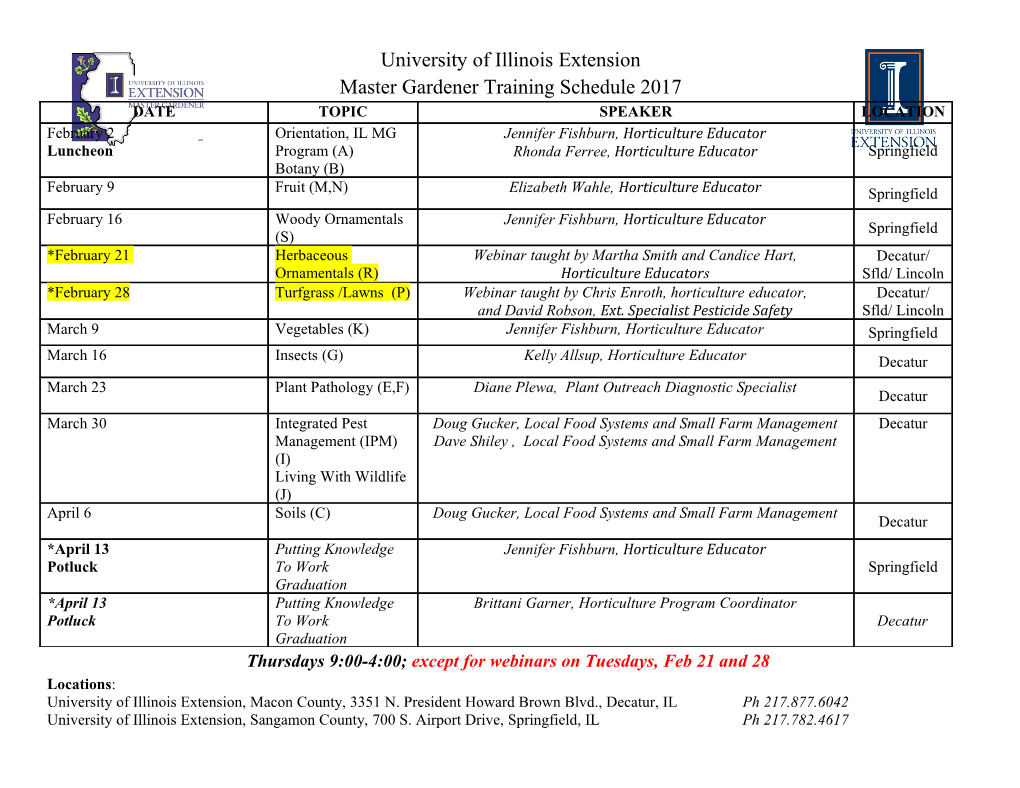
Journal of Oceanography, Vol. 55, pp. 247 to 256. 1999 Effects of Eddy Variability on the Circulation of the Japan/ East Sea 1 1 2 G. A. JACOBS , P. J. HOGAN AND K. R. WHITMER 1Naval Research Laboratory, Stennis Space Center, Mississippi, U.S.A. 2Sverdrup Technology, Inc., Stennis Space Center, Mississippi, U.S.A. (Received 5 October 1998; in revised form 17 November 1998; accepted 19 November 1998) The effect of mesoscale eddy variability on the Japan/East Sea mean circulation is Keywords: examined from satellite altimeter data and results from the Naval Research Laboratory ⋅ Japan Sea, Layered Ocean Model (NLOM). Sea surface height variations from the Geosat-Exact ⋅ eddies, ⋅ altimeter, Repeat Mission and TOPEX/POSEIDON altimeter satellites imply geostrophic velocities. ⋅ At the satellite crossover points, the total velocity and the Reynolds stress due to numerical model- ing, geostrophic mesoscale turbulence are calculated. After spatial interpolation the momentum ⋅ Reynolds stress. flux and effect on geostrophic balance indicates that the eddy variability aids in the transport of the Polar Front and the separation of the East Korean Warm Current (EKWC). The NLOM results elucidate the impact of eddy variability on the EKWC separation from the Korean coast. Eddy variability is suppressed by either increasing the model viscosity or decreasing the model resolution. The simulations with decreased eddy variability indicate a northward overshoot of the EKWC. Only the model simulation with sufficient eddy variability depicts the EKWC separating from the Korean coast at the observed latitude. The NLOM simulations indicate mesoscale influence through upper ocean–topographic coupling. 1. Introduction Lie et al., 1995). The eddy variability has been observed The Japan/East Sea (JES) general circulation is forced through analysis of sea surface temperature (SST) data primarily by the inflow through the Tsushima Strait from the (Toba et al., 1984; Miyao, 1994). The length and time scales East China Sea and by the outflow through the Tsugaru and of the eddy field have been well characterized through these Soya Straits. Tsushima Island divides the strait into east and studies. The propagation of individual eddies has also been west channels. The total transport through the strait varies documented through the SST studies (Isoda and Saitoh, significantly in time, as does the transport distribution 1993; An et al., 1994; Isoda, 1994). Using SST to perform between the eastern and western branches. The main flow an evaluation of turbulence generated by the eddy field in occurs through the west channel with a smaller portion the JES, Toba et al. (1984) demonstrate that the synoptic through the east channel. The west channel flow enters the JES circulation is dominated by mesoscale eddies. The JES as the East Korean Warm Current (EKWC) and generally mean flow is visible only after averaging over long time separates from the Korean coast between 37° and 39°N. The periods, suggesting a strong influence of the eddy field on east channel flow enters the JES as the Nearshore Branch the mean flow. and generally follows the Japanese coast to the Tsugaru In pursuit of the same goal, we use two tools to attempt Strait. Wind stress also plays a part in the JES circulation to understand eddy variability effects that occur in the JES. with the wind stress curl aiding in generating the cyclonic In this study we use sea surface height (SSH) data from the circulation north of the front. Preller and Hogan (1998) Geosat-Exact Repeat Mission (Geosat-ERM) and TOPEX/ present a review of the historical research in the JES. POSEIDON (T/P) altimeters. Measuring the SSH, the al- Particularly ubiquitous in the JES is the mesoscale eddy timeter provides geostrophic speed estimates in a direction field. perpendicular to the satellite ground track. At points where In situ eddy observations are abundant throughout the each altimeter’s ground track crosses itself, the two cross- JES (Toba et al., 1984; Ichiye and Takano, 1988; Isoda and track estimates of geostrophic velocity may be transformed Saitoh, 1993; An et al., 1994; Isoda, 1994; Lie et al., 1995; into eastward and northward velocity components. From Shin et al., 1995). Eddies have been observed extensively these velocity components, the Reynolds stresses are esti- through temperature measurements off the Korean coast in mated at crossover points (Parke et al., 1987). Gradients of the Ullung basin (Isoda and Saitoh, 1993; An et al., 1994; the Reynolds stresses produce a force on the mean flow, and 247 Copyright The Oceanographic Society of Japan. the effects of this force may be examined through a balance Hurlburt (1998) indicate a horizontal resolution of 1/32° is with the Coriolis force. This provides a balance similar to required to generate the interaction between the eddy field the balance between the horizontal pressure gradient and and bottom topography without the parameterization of Coriolis force, and this is one method to understand the topostress. Using this resolution, we examine two mecha- relative importance of the geostrophic turbulence. nisms through which the mesoscale field may influence the Numerical models provide a useful capability to add mean circulation. Both the Reynolds stresses and the upper and remove dynamical processes and then examine the ocean–topographic coupling indicate influence on the mean. effects. Hogan and Hurlburt (1998) investigated the dynamics In this examination, we compute geostrophic velocities of the JES in a progressive fashion using the Naval Research from both the Geosat-ERM and T/P satellites at each of their Laboratory’s (NRL) Layered Ocean Model (NLOM). In this respective crossover points (Section 2). The numerical model study we use the NLOM with horizontal grid resolution of experiments of the isopycnal model then demonstrate the 1/32° (3.5 km) to simulate the mesoscale variability. To effects of eddy variability on the mean circulation (Section contrast the circulation with eddy variability to the circulation 3). The results are discussed in Section 4. with reduced variability, we perform simulations with higher eddy viscosity that suppresses the eddy variability. At least 2. Altimeter Data Sets and Reynolds Stresses 1/32° resolution is necessary to resolve the mesoscale fea- This study uses altimeter data from the T/P and Geosat- tures sufficiently to ensure upper ocean–topographic coupling ERM satellites. T/P data provided on the merged Geophysical in the JES (Hogan and Hurlburt, 1998). In one 1/32° ex- Data Records (GDRs) from the Physical Oceanography periment, the eddy viscosity is set to a relatively large value Distributed Active Archive Center (PO-DAAC) over the to intentionally suppress the mesoscale eddy field (experi- time period 1993–1997 are first broken into arcs composed ment A_High). The other 1/32° experiment uses a more of one satellite revolution. Arcs that fall on the same ground realistic value of eddy viscosity and contains much more track are grouped into a set of repeat (or collinear) passes. energy in the eddy field (experiment A_Low). The mean Computed orbits for T/P are based on the Joint Gravity circulation based on the two simulations represents the Model (JGM)-3 gravity field (Tapley et al., 1996). Atmo- effects of the mesoscale variability on the mean flow. spheric corrections (dry troposphere, wet troposphere, and At low horizontal model grid resolution, the EKWC ionosphere) are applied, as well as solid Earth tides, an typically separates from the Korean coast at a latitude ocean tide estimate from the Grenoble model (Le Provost et further north than the observed separation latitude (an over- al., 1994), tidal loading, an electromagnetic (EM) bias, and shoot). Sueng and Kim (1993) indicate that the EKWC an inverse barometer correction based on the local instanta- overshoot problem is diminished (but not eliminated) by neous barometric pressure. increasing horizontal resolution to 1/5° in the Cox (1984) The National Oceanic and Atmospheric Administration ocean model. One effect of the increased model resolution (NOAA) provides the Geosat-ERM data. The orbit solutions is to better resolve the mesoscale field, and thus the model in the Geosat-ERM altimeter data set are also based on will represent the eddy field more accurately. The interac- JGM-3. Atmospheric corrections (dry troposphere, wet tion of the eddy field with the bottom topography has been troposphere, and ionosphere from the International Refer- indicated in several JES in situ studies (Toba et al., 1984; An ence Ionosphere 1995, IRI95) are applied, as well as solid et al., 1994). and ocean tide estimates (Le Provost et al., 1994), an EM Holloway et al. (1995) use the Modular Ocean Model bias correction of 2.5% of the significant wave height (MOM) at 1/5° resolution with the addition of topostress to (Witter and Chelton, 1991), and a static inverse barometer understand the effects of seasonality, wind stress, buoyancy correction. forcing, latitudinal variations in Coriolis parameter, and SSH from all repeat passes is interpolated along ground bottom interaction in the JES. The topostress parameterizes tracks to points spaced by 1 s (i.e., about 6.5 km along track), the topographic influence on the circulation (Holloway, and the mean SSH at each point along the ground tracks is 1992). Without topostress, the model of Holloway et al. subtracted removing both the geoid signal and the mean (1995) indicates the EKWC separating from the coast at a dynamic topography. The T/P data covers 5 years while the latitude further north than observed (similar to Sueng and Geosat-ERM covers only about 2.5 years. The mean SSH Kim, 1993). The topostress has the effect of increasing the removed from each satellite’s data set is the mean over its strength of the North Korean Cold Current (NKCC), which own time period. There are about 65 measurements at each causes the EKWC to separate closer to the observed latitude.
Details
-
File Typepdf
-
Upload Time-
-
Content LanguagesEnglish
-
Upload UserAnonymous/Not logged-in
-
File Pages10 Page
-
File Size-