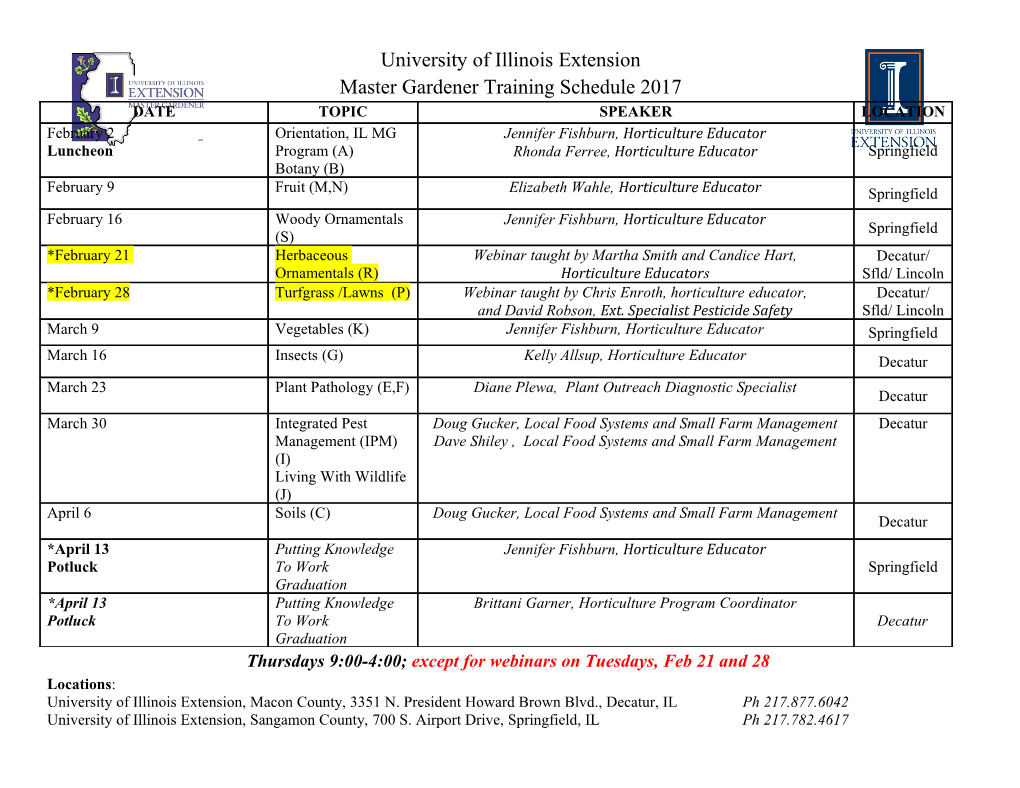
Crystal structure of mammalian selenocysteine- dependent iodothyronine deiodinase suggests a peroxiredoxin-like catalytic mechanism Ulrich Schweizera,1,2, Christine Schlickerb,1, Doreen Brauna, Josef Köhrlec, and Clemens Steegbornd,2 aInstitut für Biochemie und Molekularbiologie, Rheinische Friedrich Wilhelms-Universität Bonn, 53115 Bonn, Germany; bDepartment of Physiological Chemistry, Ruhr University Bochum, 44801 Bochum, Germany; cInstitut für Experimentelle Endokrinologie, Charité-Universitätsmedizin Berlin, 13353 Berlin, Germany; and dDepartment of Biochemistry and Research Center for Bio-Macromolecules, University of Bayreuth, 95440 Bayreuth, Germany Edited* by Dieter Söll, Yale University, New Haven, CT, and approved June 9, 2014 (received for review December 23, 2013) Local levels of active thyroid hormone (3,3′,5-triiodothyronine) are of Mus musculus Dio3 (Dio3cat)withSec170 replaced by cysteine controlled by the action of activating and inactivating iodothyro- (Fig. 1 B and C and Table 1). The structure was solved by selenium nine deiodinase enzymes. Deiodinases are selenocysteine-depen- single-wavelength anomalous dispersion phasing using crystals of cat dent membrane proteins catalyzing the reductive elimination of selenomethionine (SeMet)-labeled Dio3 . Refinement of native cat iodide from iodothyronines through a poorly understood mecha- and SeMet-labeled Dio3 resulted in structures without recog- nism. We solved the crystal structure of the catalytic domain of nizable differences, neither for the backbone (rmsd for 186 Cα mouse deiodinase 3 (Dio3), which reveals a close structural simi- positions = 0.2 Å) nor for side chains, with slightly better statistics larity to atypical 2-Cys peroxiredoxin(s) (Prx). The structure suggests for the SeMet structure (Table 1). Further analyses were thus done a route for proton transfer to the substrate during deiodination and with the SeMet structure refined at a resolution of 1.9 Å to R/Rfree a Prx-related mechanism for subsequent recycling of the transiently values of 18.7% and 22.7%, respectively. oxidized enzyme. The proposed mechanism is supported by bio- cat chemical experiments and is consistent with the effects of muta- Overall Deiodinase Structure and Substrate Binding Site. Dio3 tions of conserved amino acids on Dio3 activity. Thioredoxin and adopts a thioredoxin (Trx) fold (6) as previously predicted (7), glutaredoxin reduce the oxidized Dio3 at physiological concentra- with deiodinase-specific modifications and insertions, resulting BIOCHEMISTRY tions, and dimerization appears to activate the enzyme by displac- in architecture with a five-stranded, mixed β-sheet flanked by ing an autoinhibitory loop from the iodothyronine binding site. four α-helices (Fig. 1 B and C). N-terminal to the Trx βαβ-motif Deiodinases apparently evolved from the ubiquitous Prx scaffold, lies a small, two-stranded, antiparallel β-sheet, βN, followed by Θ and their structure and catalytic mechanism reconcile a plethora of a short 310-helix, 1. The essential deiodinase-specific insertion partly conflicting data reported for these enzymes. (Dio-insertion, residues 201–225; Fig. 1 B and C) forms a large loop-D, followed by a helix αD and a short βD that aligns with β iodothyronine deiodination | thioredoxin fold | selenoprotein | the central mixed -sheet. The loop, which is critical for iodo- thiol cofactor | selenenyl-sulfide thyronine binding and was previously called an iduronidase-like insertion, forms a compact protrusion instead of the predicted hyroid hormones regulate mammalian development as well Tas energy expenditure and metabolism in the adult (1). The Significance active, nuclear receptor-binding form of thyroid hormone is Deiodinases activate and inactivate thyroid hormones through 3,3′,5-triiodothyronine (T3). The thyroid gland mainly releases a unique biochemical reaction. Enzymes expand their catalytic thyroxine [3,3′,5,5′-tetraiodothyronine (T4); Fig. 1A], and T3 is formed and degraded through elimination of iodine atoms from capabilities through special heteroatoms in cofactors or in the the 5′- and 5-positions, respectively (2) (Fig. S1). Three types of rare but essential amino acid selenocysteine, and deiodinases deiodinase enzymes are involved in activation and inactivation use an active-site selenocysteine for the reductive elimination of thyroid hormones (Fig. S1). They form a family of trans- of iodide from the aromatic iodothyronine rings. The mecha- membrane enzymes with homologous catalytic domains (2). nism of deiodinases has remained elusive despite many mu- Type II deiodinase (Dio2) catalyzes the activating (outer ring) tational and enzymatic studies. We solved the crystal structure of the deiodinase catalytic domain and find that it resembles 5′-deiodination of the prohormone T4 to T3, whereas type III deiodinase (Dio3) catalyzes inactivating (inner ring) 5-deiodination a family of peroxiredoxin(s) (Prx). Structure and biochemical (2). Type I deiodinase (Dio1) is capable of both types of reac- data suggest a deiodinase catalytic mechanism with Prx-like tions (3). Cells targeted by the hormone can thereby fine-tune elements and enable us to assign unexpected functions to residues previously reported to contribute to deiodinase ca- intracellular T3 levels through deiodinase expression according to their needs during development or upon metabolic challenges. talysis. Our findings indicate how deiodinases may have evolved Dio1, Dio2, and Dio3 share a conserved amino acid sequence from a common reductase ancestor. and a selenocysteine residue essential for efficient deiodination, Author contributions: U.S. and C. Steegborn designed research; C. Schlicker and D.B. although nonmammalian cysteine-deiodinases have been found performed research; U.S., C. Schlicker, D.B., J.K., and C. Steegborn analyzed data; and (4, 5). Although most of the characterized selenoproteins act U.S., C. Schlicker, D.B., J.K., and C. Steegborn wrote the paper. as peroxidases or protein reductases, deiodinases are the only The authors declare no conflict of interest. selenoenzymes known to catalyze halogen eliminations from *This Direct Submission article had a prearranged editor. aromatic rings. Despite a large body of experimental data, there Data deposition: The atomic coordinates and diffraction data have been deposited in the is no coherent model for deiodinase catalysis that incorporates Protein Data Bank, www.pdb.org (PDB ID codes 4TR3 and 4TR4). the bulk of available data. 1U.S. and C. Schlicker contributed equally to this work. 2To whom correspondence may be addressed. Email: [email protected] or clemens. Results and Discussion [email protected]. To explore the unique deiodinase catalytic mechanism, we de- This article contains supporting information online at www.pnas.org/lookup/suppl/doi:10. termined the crystal structure of the cytoplasmic catalytic domain 1073/pnas.1323873111/-/DCSupplemental. www.pnas.org/cgi/doi/10.1073/pnas.1323873111 PNAS Early Edition | 1of6 Downloaded by guest on September 25, 2021 Fig. 1. Crystal structure of Dio3cat.(A) Chemical structure of T4 with numbering of iodine positions (underlined). (B) Overall structure of the Dio3 catalytic domain, with sec- ondary structure elements present in Trx in cyan and la- beled according to convention. The N-terminal Prx-like module is highlighted in magenta, the Dio-insertion is highlighted in blue, and α2 is highlighted in green. (C) Dio3cat topology diagram. Catalytically relevant amino acids are labeled. (D) Active site region of Dio3cat, with residues conserved and/or involved in catalysis shown in stick presentation. Sec170* indicates the catalytic seleno- cysteine replaced by cysteine in our construct. (E) Close-up view of a model for Dio3 in complex with iodothyronine ligand, based on the Arg-T3-His clamp in T3Rβ (Dio3 sub- strate would be T4). (F) 5-Deiodinase activity of WT Dio3 and mutants with changes in the α2/β3- and β4/α3-loops in the presence of DTT. Error bars indicate SEM (n = 3). α-helix (7). The catalytic Sec170 (replaced by a cysteine in our Dio3-His202, which corresponds to the catalytically essential Dio1- construct, as indicated by *) is positioned in the loop connecting His158, protrudes from the Dio-insertion into the substrate binding β1toα1, in the same position as the peroxidatic cysteine of thiol cleft (Fig. 1D). Its distance to the catalytic selenocysteine and reductases with a Trx-like fold [Trx, peroxiredoxins (Prx), and position at the end of this cleft suggest that rather than acting glutathione (GSH) peroxidases GPX (see below); Fig. 1D]. It catalytically, His202 serves as a binding partner for the 4′-phe- 435 points toward an elongated cleft that likely represents the iodo- nolic end of the substrate, similar to His in the T3-receptor β 435 282 thyronine binding site. Two conserved deiodinase histidines are (T3Rβ) complex (9). Superimposing the His -T3-Arg clamp 158 174 202 275 important for Dio1 activity, His and His (8). The side chain from the T3/T3Rβ complex on Dio3-His -Arg provides a first of Dio3-His219 (corresponding to Dio1-His174) is buried between substrate complex model and places the iodothyronine into the loop-D and helix αD and appears to contribute to catalysis proposed Dio3 substrate binding cleft (Fig. 1E). The targeted 5- through an interaction with the conserved Glu200 (see below). iodine atom is positioned ∼3–4 Å from the Se atom of Sec170, Table 1. Data collection and refinement statistics SeMet Dio3 Native Dio3 Space group P212121 P212121 Unit
Details
-
File Typepdf
-
Upload Time-
-
Content LanguagesEnglish
-
Upload UserAnonymous/Not logged-in
-
File Pages6 Page
-
File Size-