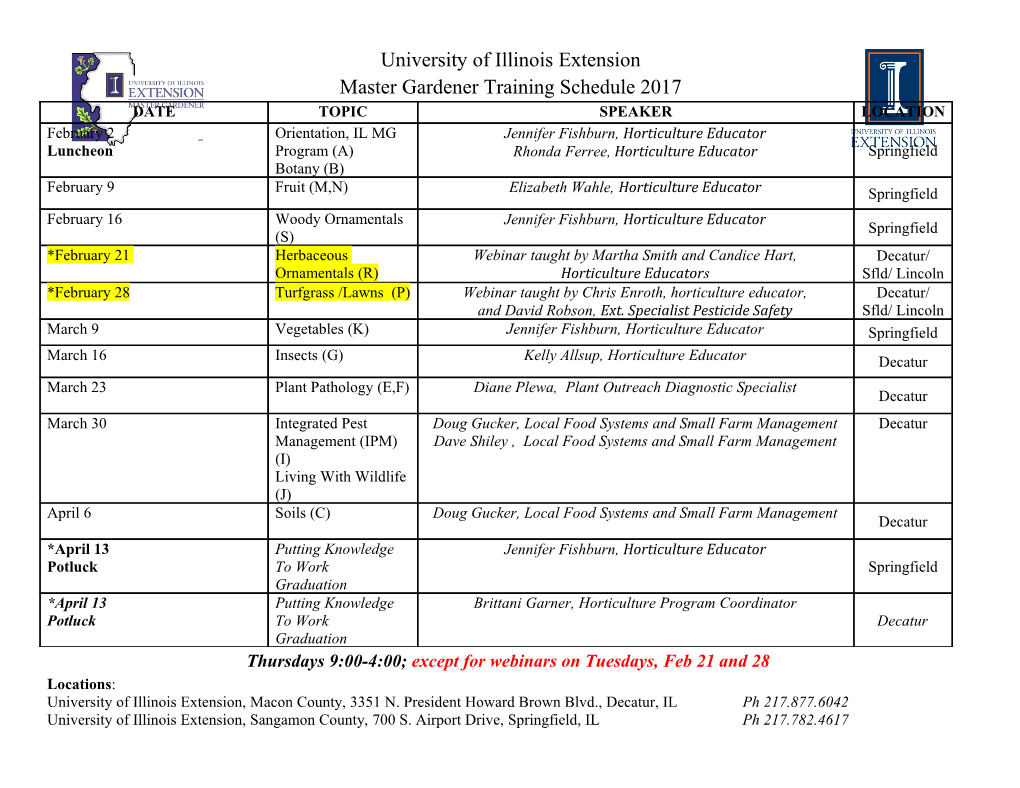
INTERNATIONAL SOCIETY FOR SOIL MECHANICS AND GEOTECHNICAL ENGINEERING This paper was downloaded from the Online Library of the International Society for Soil Mechanics and Geotechnical Engineering (ISSMGE). The library is available here: https://www.issmge.org/publications/online-library This is an open-access database that archives thousands of papers published under the Auspices of the ISSMGE and maintained by the Innovation and Development Committee of ISSMGE. Paper No. LIUTR LIQUEFACTION-INDUCED UPLIFT OF BURIED STRUCTURES INSIGHTS FROM THE STUDY OF AN IMMERSED RAILWAY TUNNEL Thaleia TRAVASAROU 1, Weiyu CHEN2, and Jacob CHACKO3 ABSTRACT Light buried structures surrounded by liquefiable soils are susceptible to uplift during large earthquakes. Liquefaction-induced uplift, often referred to as “floatation”, has been commonly associated with significant displacements and is usually considered the result of a force-based buoyancy mechanism. Resistance to such mechanism is provided by the available shear strength of the liquefiable soil and a reverse end bearing capacity. We explored the mechanism of uplift in the context of the vulnerability and retrofit studies for an existing 5.8-km long immersed railroad tunnel located 15 km from the San Andrea fault in the Bay Area of California. The tunnel, built in the 1960’s, was placed in a trench excavated into the native soils and backfilled with loose gravel and sand. Two-dimensional effective stress dynamic soil- structure-interaction analyses complemented by two project-specific centrifuge experiments estimated relatively small tunnel uplift. Based on the analyses results and experimental observations we concluded that the governing mechanism of uplift is a displacement-limited ratcheting phenomenon associated primarily with co-seismic movement of soil under the tunnel. The amount of uplift is related primarily to the extent of liquefiable soils, their relative density, the differential weight between the buried structure and surrounding soil and amplitude and duration of shaking. Additional, sources are related to volumetric expansion of soil due to water inflow and potential heaving of soft soils. The methodology developed to assess the uplift vulnerability for this critical structure led to an improved understanding of liquefaction- induced uplift and the adoption of a no-retrofit strategy with significant cost savings. It also provides a framework for the future assessment of liquefaction-induced uplift vulnerability for a range of structures. Keywords: Liquefaction, immersed tunnel, uplift, centrifuge, soil-structure-interaction, numerical analyses OVERVIEW OF LIQUEFACTION-INDUCED UPLIFT Uplift of light structures buried in liquefiable soils has been observed during large earthquakes (e.g., Seed, 1967; Koseki, Matsuo, Ninomiya, & Yoshida, 1997; Yasuda and Kiku, 2006), and reported during shaking table tests (e.g., Koseki et al., 1997; Yasuda et al., 1994; Tamura et al., 1997), and centrifuge tests (e.g., Adalier et al. 2002 and 2003, Sasaki and Tamura, 2004). In most of the cases involving strong shaking large uplift (i.e., sometimes on the order of meters) was observed. As a result liquefaction- induced uplift has traditionally been referred to as “floatation” (e.g., Seed, 1967; Tamura et. al., 1997; Yasuda et al., 1994) suggesting a mechanism related to equilibrium of vertical forces. This concept implies that during strong shaking the soil liquefies fully (i.e., 100 percent excess pore pressure) turning into a viscous fluid and imparting upward buoyancy forces on the buried structure. Hence some researchers proposed (e.g., Koseki et. al., 1997; Tamura et. al., 1997) that uplift vulnerability be assessed using limit equilibrium stability analyses. We refer to this force-equilibrium involving the assumption of buoyancy as the “force-based” concept for the uplift mechanism. This concept implies that uplift occurs 1 Senior Engineer, Earthquake Group, Fugro West, Inc., e-mail: [email protected] 2 Senior Engineer, Earthquake Group, Fugro West, Inc. 3 Principal, Earthquake Group, Fugro West, Inc. 5th International Conference on Earthquake Geotechnical Engineering January 2011, 10-13 Santiago, Chile rapidly after soil liquefaction and is related to large displacements. However, observations from physical model tests suggest that structural uplift occurs primarily co-seismically (e.g., Sasaki and Tamura, 2004, and Koseki et al., 1997) which would be in contrast to a force-based mechanism. Until recently limited literature existed on liquefaction-induced uplift of tunnel structures. A combination of centrifuge model testing (Adalier et al. 2002 and 2003) and numerical analyses (Yang et. al., 2004, Naesgaard et al., 2004) were performed for the George Massey Tunnel in Vancouver, Canada to help guide the decision for retrofit. In this case tunnel uplift was judged to be substantial and a stone column retrofit plan was proposed to limit uplift deformations. We studied the mechanism of liquefaction-induced structural uplift in the context of the analytical and experimental work performed to assess the vulnerability to uplift and need for retrofit of the Bay Area Rapid Transit (BART) submerged railway tunnel near the city of San Francisco. At the onset of the project, there was consensus in the scientific community regarding the liquefaction susceptibility of the very loose gravels and sands surrounding the tunnel under strong design shaking. However, due to the limited data and documentation of liquefaction-induced uplift in the literature the expected consequences of soil liquefaction for the tunnel were not obvious and potential catastrophic uplift associated with a force-based mechanism could not be precluded. The extensive numerical analyses and testing performed subsequently for this project, and discussed in this paper, helped gain improved understanding of the uplift mechanism which was found to be primarily a displacement-limited ratcheting phenomenon. In the case of the BART tunnel the implications of the governing uplift mechanism estimating relatively small displacements enabled the adoption of a no-retrofit strategy with substantial cost benefits. The analyses performed for the BART tunnel project provide a framework that can be used in the future to evaluate the liquefaction-induced uplift vulnerability for a range of buried structures. BART OFFSHORE TRANSBAY TUBE PROJECT DESCRIPTION The Bay Area Rapid Transit (BART) Offshore Transbay Tube (TBT) is a 5.8 km-long immersed cut and cover railway tunnel built in the 1960s and connecting San Francisco to Oakland in the high seismicity Bay Area in California (Figure 1a). The TBT is the most important transportation infrastructure in the Bay Area carrying more than 300,000 passengers daily. It is located at close distances of about 14 km and 9 km, respectively, from the San Andreas and Hayward faults with estimated characteristic magnitudes of 7.9 and 7.25 and relatively high slip rates on the order of 17 and 9 mm/year. The project design spectra for outcropping “soft rock” corresponding approximately to a 1,000-year return period Bechtel (2005) are presented in Figure 1b and show high ground motions with peak ground acceleration of about 0.63g. The TBT was constructed in a trench excavated into the native soils (Figure 2). After trench excavation an approximately 1.2-meter-thick layer of gravel was placed and screeded level. Prefabricated segments of tube, approximately 100 meters-long each, were immersed and connected together. The equivalent unit weight of the TBT is approximately 1.1 times that of sea water. Backfill material consisting of gravel was then tremmied around the sides of the tube section to about the middle height. Sand fill material was subsequently pumped in to provide a minimum cover of about 1.5 meters above the TBT. Natural sedimentation formed a soft impermeable layer of surficial mud. The relative density of the trench backfills was inferred by 36 pairs of Cone Penetration (CPT) soundings Fugro (2007b) performed at about 1.5-meter distance from the tunnel edge on either side of the tunnel. Permeability was estimated with in-situ tests and empirical correlations based on gradation curves. Table 1 summarizes the soil properties interpreted from all available data. In both the gravel and sand fill the tip resistance is relatively uniform and low, with median values corresponding to relative density of about 5th International Conference on Earthquake Geotechnical Engineering January 2011, 10-13 Santiago, Chile 35% and 40%, respectively. The permeability of the gravel fill and foundation is large with best estimate values of 1 to 5 cm/s, while permeability in the sandy fill is approximately 100 smaller. 2.0 Matched Motions 1.6 Design Spectra 5 % Damping 1.2 0.8 0.4 Spectral Acceleration (g) 0.0 0.01 0.1 1 10 Period (s) Figure 1. a) Location of BART offshore immersed tunnel with respect to major faults, b) Project design acceleration spectrum and seven spectrally-matched time histories Surficial mud Sand fill Stiff clay Gravel fill Gravel foundation Figure 2. Typical cross-section of immersed tunnel and surrounding trench backfills Table 1. Key trench backfill properties Dr (%) Permeability (cm/s) qc1N Unit best estimate best estimate SOIL TYPE best estimate Weight N (16%-84% (16%-84% 1,60 (16%-84% percentiles) (kN/m3) percentiles) percentile) Sand Fill 50 (32-70) 40 (35-45) 0.01 (0.002-0.1) 1.9 7 Gravel Fill 33 (25-48) 35 (30-40) 1 (0.3-10) 2.1 6 Gravel 33 (25-48) 35 (30-40) 1 (0.3-10) 2.1 6 Foundation
Details
-
File Typepdf
-
Upload Time-
-
Content LanguagesEnglish
-
Upload UserAnonymous/Not logged-in
-
File Pages12 Page
-
File Size-