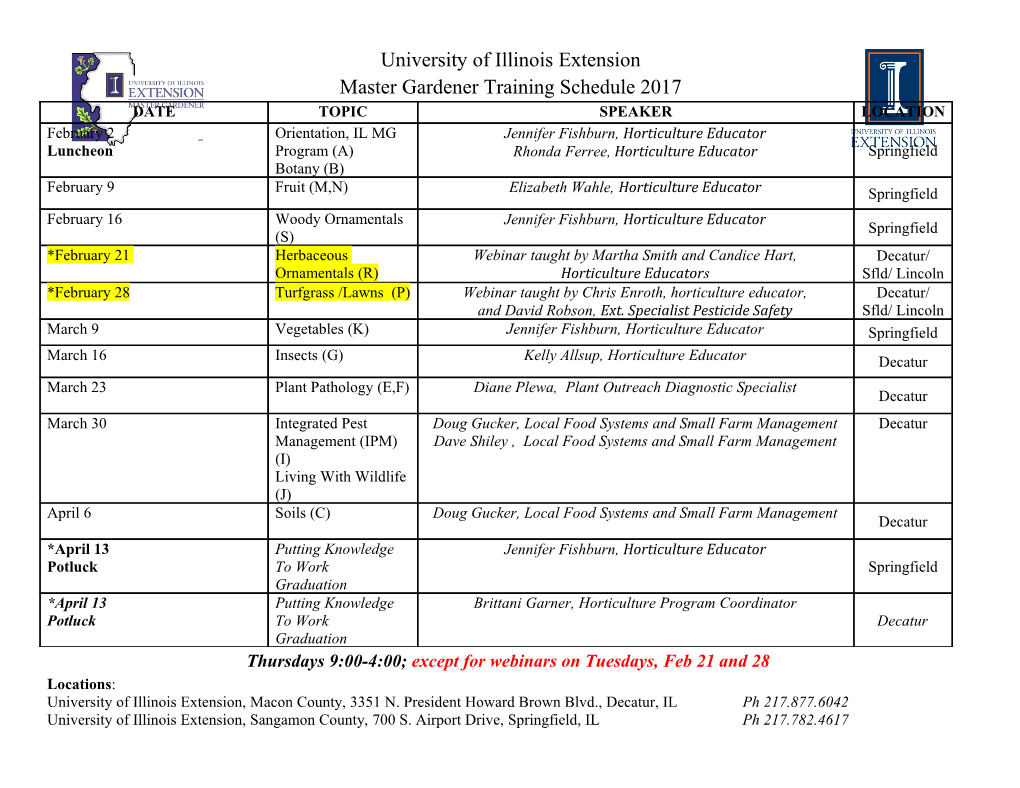
Mu transpososome and RecBCD nuclease collaborate in the repair of simple Mu insertions Wonyoung Choi1, Sooin Jang, and Rasika M. Harshey2 Department of Molecular Biosciences and Institute of Cellular and Molecular Biology, The University of Texas at Austin, Austin, TX 78712 Edited* by Nancy L. Craig, The Johns Hopkins University School of Medicine, Baltimore, MD, and approved July 31, 2014 (received for review April 24, 2014) The genome of transposable phage Mu is packaged as a linear than that the gap-filling E. coli polymerase PolA is not required segment, flanked by several hundred base pairs of non-Mu DNA. and that the double-strand break (DSB) repair machinery is The linear ends are held together and protected from nucleases by somehow involved (14). the phage N protein. After transposition into the Escherichia coli We show in this study that the noncovalently closed configu- chromosome, the flanking DNA (FD) is degraded, and the 5-bp ration of the FD in the infecting Mu donor substrate controls the gaps left in the target are repaired to generate a simple Mu in- fate of the strand-transfer joint. Our data show that the RecBCD sertion. Our study provides insights into this repair pathway. The exonuclease is required in vivo for degradation of the FD. This data suggest that the first event in repair is removal of the FD by occurs only after integration of infecting Mu, suggesting that there the RecBCD exonuclease, whose entry past the N-protein block is is a timed mechanism to remove N and allow RecBCD access into licensed by the transpososome. In vitro experiments reveal that, the FD. Attempts to recapitulate this reaction in vitro have when RecBCD is allowed entry into the FD, it degrades this DNA until it arrives at the transpososome, which presents a barrier for revealed that the FD is processed in two stages: First, RecBCD further RecBCD movement. RecBCD action is required for stimulat- degrades the long DNA, and next, a specific endonucleolytic ing endonucleolytic cleavage within the transpososome-protected cleavage leaves short 4-nt flanks within the transpososome- DNA, leaving 4-nt flanks outside both Mu ends. This end product protected DNA. Earlier, we had found that a cryptic endonu- of collaboration between the transpososome and RecBCD resem- clease activity of MuA was required for removal of the flanks in bles the intermediate products of Tn7 and retroviral and retro- vivo, and had interpreted the data to suggest that degradation of transposon transposition, and may hint at a common gap-repair this DNA is initiated by endonucleolytic cleavage (15). In light of BIOCHEMISTRY mechanism in these diverse transposons. the results obtained in the present study, we reinterpret our earlier data and propose a new model for repair. We expect that conservative integration | nonreplicative transposition this first biochemical analysis, to our knowledge, of the initial steps of Mu repair will reveal pathways common to the repair of he repair of transposon insertions is the least understood all transposon insertions. Taspect of transposon biology. Transposable phage Mu pro- vides an excellent system to study this process because of its high Results transposition frequency. Mu is a temperate phage, which uses Flanking-DNA Removal in Vivo Depends on the Nuclease Activity of transposition not only to integrate into its Escherichia coli host to RecBCD. Repair of Mu insertions involves two events: removal of generate prophages but also to amplify its genome during the lytic the FD and the filling in of the 5-bp gaps (Fig. 1B). Of these, the – cycle, where transposition is coupled to replication (Fig. 1A)(1 4). former is readily monitored, because this DNA is long. We have This study focuses on the transposition event that occurs im- therefore used this reaction as an in vivo assay for repair. mediately after infection, which is followed by repair rather than replication of the Mu insertion. The infecting Mu genome is Significance linear, and is linked to several hundred base pairs of non–Mu- flanking DNA. The tips of this DNA are held together by an injected phage protein, N, which converts it into a noncovalently Transposition is not finished until the gaps left in the target in closed circular form and protects the DNA against exonucleases the wake of transposition are repaired, yet this is the least (5–7). After integration, the flanking DNA (FD) is removed (8), understood aspect of transposition. This study describes to our and the 5-bp gaps generated in the target are repaired to yield knowledge the first in vitro assay for repair of Mu insertions, a simple Mu insertion (Fig. 1B) (9). Unlike the noncovalently which shows that repair is controlled by both transpososome closed configuration of the infecting Mu genome, replicating Mu and host factors. The long flanking DNA (FD) attached to Mu is is initially part of a covalently closed circular E. coli chromosome shortened in two stages, requiring collaboration between the (Fig. 1A). At the end of the lytic phase, Mu replicas are packaged transpososome and RecBCD at both stages. The transpososome such that host DNA linked to either side of the insertion is in- initially regulates the timing of RecBCD entry into the FD, and cluded in the phage head; this is the source of the FD in the then exploits RecBCD action on this DNA to promote endonu- cleolytic cleavage within the transpososome. The final product infecting Mu genome. has short flanks, and is likely the substrate for gap repair by The chemical steps of Mu transposition during the replication a host polymerase. and repair pathways are the same, namely the MuA transposase nicks Mu DNA at each end and then joins the nicked ends to Author contributions: W.C. and R.M.H. designed research; W.C. and S.J. performed re- target DNA cleaved 5 bp apart (Fig. 1) (2, 8). However, the search; W.C. contributed new reagents/analytic tools; W.C., S.J., and R.M.H. analyzed resulting branched strand-transfer intermediate is resolved al- data; and W.C. and R.M.H. wrote the paper. ternatively by target-primed replication during the lytic phase The authors declare no conflict of interest. (Fig. 1A) (10) and by FD removal and limited replicative repair *This Direct Submission article had a prearranged editor. during the infection phase (Fig. 1B) (8, 11). The alternate fates 1Present address: Howard Hughes Medical Institute and Department of Molecular Biology of a similar strand-transfer joint have long been a matter of and Microbiology, Tufts University School of Medicine, Boston, MA 02111. speculation. Although much has been learned about the proteins 2To whom correspondence should be addressed. Email: [email protected]. that promote the transition from transposition to replication (10, This article contains supporting information online at www.pnas.org/lookup/suppl/doi:10. 12, 13), little is known about those that assist in repair, other 1073/pnas.1407562111/-/DCSupplemental. www.pnas.org/cgi/doi/10.1073/pnas.1407562111 PNAS Early Edition | 1of6 Downloaded by guest on September 23, 2021 We conclude that the exonuclease activity of RecBCD is re- quired for removal of the FD after transposition of infecting Mu. Degradation by RecBCD Depends on the Flanking-DNA Configuration. The Mu transposition reaction is well-established in vitro (2, 3). Addition of MuA/MuB proteins and E. coli HU protein to a mini- Mu donor plasmid leads to Mu end cleavage by the MuA trans- posase, followed by MuB-assisted strand transfer of the cleaved ends into a target plasmid to generate the Ɵ-shaped strand- transfer intermediate (Fig. 3A, lane 1 and Fig. 3B, Center). When treated with SDS, the Ɵ complex disassembles to generate a se- Fig. 1. Two transposition pathways during the Mu life cycle. The chemical ries of topoisomers, reflecting supercoils still trapped within the steps of single-stranded DNA cleavage at Mu ends followed by strand Mu segment of the Ɵ structure (lane 2). Addition of RecBCD to transfer (ST) of the cleaved ends to phosphodiester bonds spaced 5 bp apart Ɵ on the target are the same in both the lytic (A) and infection (B) phases of the complex did not change its configuration (lanes 3 and 4). transposition. During the lytic phase, Mu transposition is intramolecular, and Although ClpX is required in vivo for efficient removal of the the ST intermediate is resolved by target-primed replication through Mu (A). FD (Fig. S1) (15), addition of ClpX did not promote degradation During the infection phase, transposition is intermolecular, and the Ɵ ST of the Ɵ complex by RecBCD. intermediate is resolved by removal of the flanking DNA and repair of the RecBCD requires a free double-stranded DNA end for entry 5-bp gaps left in the target (B). The FD is covalently closed in A but non- (21). Such an end is not available on the Ɵ complex in vitro, but is covalently closed by phage N protein (oval) bound to the tips of the FD in B. potentially available in the Ɵ complex in vivo if the N protein were The target DNA flanking Mu is red in all of the figures. Arrowheads indicate to be removed (Fig. 3B). To mimic such an in vivo substrate, the the 3′ ends. FD on the in vitro Ɵ complex was linearized with the restriction enzyme XmnI (Fig. 3B, Center); the XmnI cut site was positioned An earlier genetic screen designed to identify host factors to leave 250 bp and 2 kb of DNA outside the Mu L end and Mu R required for posttransposition recovery (and hence repair) of Mu end, respectively, approximating the normal Mu FD lengths.
Details
-
File Typepdf
-
Upload Time-
-
Content LanguagesEnglish
-
Upload UserAnonymous/Not logged-in
-
File Pages6 Page
-
File Size-