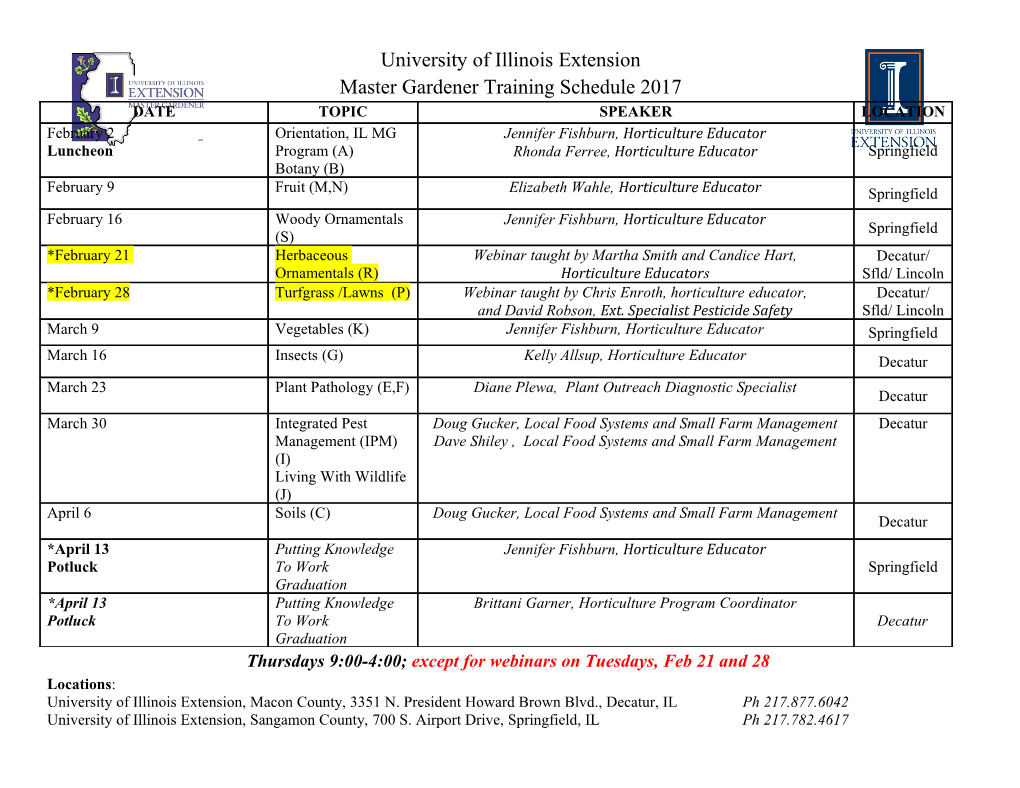
UCRL-CONF-222137 SHOCK INITIATION EXPERIMENTS AND MODELING OF COMPOSITION B AND C-4 Paul A. Urtiew, Kevin S. Vandersall, Craig M. Tarver, Frank Garcia, Jerry W. Forbes June 16, 2006 13th International Detonation Symposium Norfolk, VA, United States July 23, 2006 through July 28, 2006 Disclaimer This document was prepared as an account of work sponsored by an agency of the United States Government. Neither the United States Government nor the University of California nor any of their employees, makes any warranty, express or implied, or assumes any legal liability or responsibility for the accuracy, completeness, or usefulness of any information, apparatus, product, or process disclosed, or represents that its use would not infringe privately owned rights. Reference herein to any specific commercial product, process, or service by trade name, trademark, manufacturer, or otherwise, does not necessarily constitute or imply its endorsement, recommendation, or favoring by the United States Government or the University of California. The views and opinions of authors expressed herein do not necessarily state or reflect those of the United States Government or the University of California, and shall not be used for advertising or product endorsement purposes. SHOCK INITIATION EXPERIMENTS AND MODELING OF COMPOSITION B AND C-4 Paul A. Urtiew1, Kevin S. Vandersall1, Craig M. Tarver1, Frank Garcia1, and Jerry W. Forbes2 1Energetic Materials Center, Lawrence Livermore National Laboratory, Livermore, CA 94550 2Center for Energetic Concepts Development, University of Maryland, College Park, MD 20742 Abstract. Shock initiation experiments on the explosives Composition B and C- 4 were performed to obtain in-situ pressure gauge data for the purpose of determining the Ignition and Growth reactive flow model with proper modeling parameters. A 101 mm diameter propellant driven gas gun was utilized to initiate the explosive charges containing manganin piezoresistive pressure gauge packages embedded in the explosive sample. Experimental data provided new information on the shock velocity versus particle velocity relationship for each of the investigated materials in their respective pressure range. The run-distance- to-detonation points on the Pop-plot for these experiments showed agreement with previously published data, and Ignition and Growth modeling calculations resulted in a good fit to the experimental data. These experimental data were used to determine Ignition and Growth reactive flow model parameters for these explosives. Identical ignition and growth reaction rate parameters were used for C-4 and Composition B, and the Composition B model also included a third reaction rate to simulate the completion of reaction by the TNT component. The Composition B model was then tested on existing short pulse duration, gap test, and projectile impact shock initiation with good results. This Composition B model can be applied to shock initiation scenarios that have not or cannot be tested experimentally with a high level of confidence in its predictions. INTRODUCTION of particular interest for reasons of safety and understanding of their behavior under dynamic Shock initiation is one of the most important loading conditions. properties of energetic materials (EM), which In earlier publications we have reported determines whether or not the material will build initiation thresholds for build up to detonation up in pressure after being shocked and eventually and sensitivity to impact (Pop-plots) of both transit to a full-fledged detonation or if it will die sensitive and insensitive HMX and TATB based out before it gains strength from induced explosives at various initial temperatures.1,2 In reaction. Study of this property is important to this publication we will report on initiation and gain knowledge of when the material will sensitivity of more common RDX based detonate as intended when intentionally shocked explosives known as C-4 and Composition B. and, at the same time, when it will not detonate This work will provide previously unpublished when it is accidentally exposed to dynamic manganin gauge records for sustained pulse loading. shock initiation and will compliment it with Energetic materials are widely used in both modeling short shock pulse shock initiation industrial applications and at defense oriented experiments by Trott and Jung;3 embedded establishments. However, some of the most particle velocity gauge detonation experiments common energetic materials are also used for ill by Cowperthwaite and Rosenberg;4 and failure intent. Therefore, initiation of such materials is diameter / detonation wave curvature experiments by Campbell and Engelke.5 This velocity. The intersection of these two lines is reactive flow model uses one reaction growth taken as the “run-distance-to-detonation,” which rate for the RDX component of Composition B is then plotted on the “Pop-Plot” showing the and another reaction growth rate for the TNT. run-distance-to-detonation as a function of the Similar models for mixtures, such RX-26-AF, input pressure in log-log space. which is approximately half HMX and half Single TATB, have been used in this manner Element successfully.6 Gauges EXPERIMENTAL TECHNIQUE Velocity & Tilt Crystal Pins The Shock Initiation experiments were performed on the 101 mm bore, propellant driven gas gun, which allows precise control of Flyer Disc Target the projectile velocity and of the loading pressure Plate Sabot imposed on the energetic material target. The Single experimental set-up is illustrated in Fig 1. Element Gauges There were two types of target assemblies that were used in these experiments. One target Velocity & Tilt assembly consisted of several discs of different Crystal Pins thicknesses. Gauge packages containing manganin pressure gauges were embedded Multiple Element between individual discs. The other target Gauge assembly consisted of two 24˚ wedges with one Wedge Target multi-element pressure gage package placed Figure 1. Schematic diagram of experimental between them. The intent here was to eliminate set-up for gun experiments. the effect of inert package material on each consecutive gauge element. The gauges are Table 1. Listing of Shock Initiation Gun armored with thin (125 µm) Teflon insulation on Experiments both sides to prevent shorting of the gauges in a Shot Target Velocity Pressure Dist. to conductive medium when the material becomes # (km/s) (GPa) Det. (mm) reactive. Other details of the manganin pressure 4565 C-4 0.600 2.20 25 gauges are described in our previous 4547 C-4 0.737 2.86 17 publications.7,8 4564 C-4 0.987 4.20 9 For better control of the impact pressure, a 4359 Comp. B 0.835 3.78 16 thin buffer plate of the same material as the 4544 Comp. B 0.929 4.35 12 impact plate is placed in front of the target assembly for symmetrical impact. Also included 4540 Comp. B 1.005 4.80 10 in the two target assemblies are six tilt pins 4545 Comp. B 1.307 6.84 5 placed around the periphery of the target flush with the impact surface to measure the tilt of the EXPERIMENTAL RECORDS impact plate as it strikes the target, and four velocity pins sticking out some known distance A total of 7 shots were fired of which 3 of from the target to measure the velocity of the them were with the C-4 explosives in the disc impact plate just before it strikes the target. target configuration and 4 with the Composition During the experiment, oscilloscopes B in the wedge target configuration. Impact measure change of voltage as result of resistance velocities and pressures imposed on the targets change in the gauges which were then converted material are listed in Table 1. to pressure using the hysteresis corrected Figure 2 shows pressure records of a shock calibration curve published elsewhere.9 From the loaded RDX-based high explosive C-4 (91 data of the shock arrival times of the gauge weight % RDX and 9 weight % of other locations, a plot of distance vs. time (“x-t plot”) additives) pressed to 98.5% of theoretical is constructed with the slope of the plotted lines maximum density. The explosive samples in yielding the shock velocities with two lines these experiments were shock loaded with an apparent, a line for the un-reacted state as it aluminum impactor flying at a velocity of 0.6, reacts and a line representing the detonation mm/µs, imposing a pressure on the target material 2.2 GPa. As in all heterogeneous 100 7 Pop Plot for C-4 and Comp. B 6 explosives these traces exhibit the characteristic at ambient conditions 5 features of their initiation: some reaction occurs 4 3 LX17 amb. just behind the shock front causing it to grow in PBX 9404 2 Comp B (G & P) C-4 (Experiments) pressure, but most of the reaction occurs well (G & P) Comp B (Experiments) behind the leading shock, creating a pressure 10 7 wave that overtakes the initial shock wave 6 5 causing the process to finally transit to 4 3 detonation. In this case the transition to 2 Run Distance to Detonation (mm) LX-17 amb. detonation occurred at about 25 mm into the PBX 9404 1 target. 2 3 4 5 6 7 8 9 2 3 4 5 6 7 8 9 Figure 3 illustrates the records obtained with 1 10 100 Impact Pressure (GPa) a wedge type experiments performed on another Figure 4. Pop-Plot showing data from C-4 and RDX based explosive Composition B (63-weight Composition B along with reference lines. % RDX, 36-weight % TNT and 1weight % of Wax) at ambient room temperature. It shows Shock sensitivity of both C-4 and initially a steady shock wave and then, after the Composition B for various initial pressures is reaction becomes significant, a strong growth in illustrated in Fig. 4 on a so-called “Pop Plot”, pressure just before the transition to detonation.
Details
-
File Typepdf
-
Upload Time-
-
Content LanguagesEnglish
-
Upload UserAnonymous/Not logged-in
-
File Pages12 Page
-
File Size-