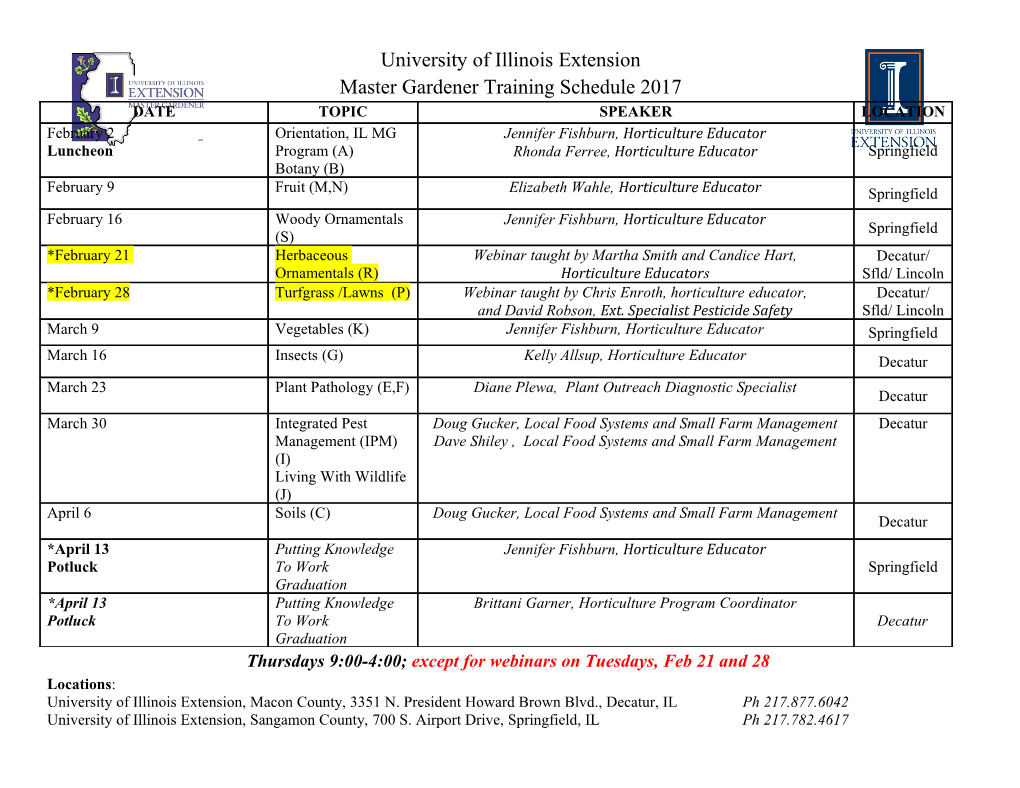
中国科技论文在线 http://www.paper.edu.cn Comparative Biochemistry and Physiology, Part D 3 (2008) 96–107 Molecular evolution of the vertebrate hexokinase gene family: Identification of a conserved fifth vertebrate hexokinase gene ⁎ David M. Irwin a, , Huanran Tan b a Department of Laboratory Medicine and Pathobiology, Banting and Best Diabetes Centre, University of Toronto, Toronto, Canada b Department of Pharmacology, Peking University Health Sciences Centre, Beijing, China Received 6 October 2007; received in revised form 12 November 2007; accepted 13 November 2007 Available online 3 December 2007 Abstract Hexokinases (HK) phosphorylate sugar immediately upon its entry into cells allowing these sugars to be metabolized. A total of four hexokinases have been characterized in a diversity of vertebrates—HKI, HKII, HKIII, and HKIV. HKIV is often called glucokinase (GCK) and has half the molecular weight of the other hexokinases, as it only has one hexokinase domain, while other vertebrate HKs have two. Differing hypothesis has been proposed to explain the diversification of the hexokinase gene family. We used a genomic approach to characterize hexokinase genes in a diverse array of vertebrate species and close relatives. Surprisingly we identified a fifth hexokinase-like gene, HKDC1 that exists and is expressed in diverse vertebrates. Analysis of the amino acid sequence of HKDC1 suggests that it may function as a hexokinase. To understand the evolution of the vertebrate hexokinase gene family we established a phylogeny of the hexokinase domain in all of the vertebrate hexokinase genes, as well as hexokinase genes from close relatives of the vertebrates. Our phylogeny demonstrates that duplication of the hexokinase domain, yielding a HK with two hexokinase domains, occurred prior to the diversification of the hexokinase gene family. We also establish that GCK evolved from a two hexokinase domain-containing gene, but has lost its N-terminal hexokinase domain. We also show that parallel changes in enzymatic function of HKI and HKIII have occurred. © 2007 Elsevier Inc. All rights reserved. Keywords: Glucokinase; Gene duplication; Hexokinase; Gene evolution 1. Introduction 1995, 2003; Cárdenas et al., 1998). Hexokinase IV is more often called glucokinase (GCK) although it does not differ in its Phosphorylation of glucose by ATP occurs immediately specificity to glucose compared to the other hexokinases upon its import into eukaryotic cells, with the phosphorylation (Wilson, 1995, 2003; Cárdenas et al., 1998). Most hexokinases being mediated by hexokinases (HK) (Wilson, 1995, 2003; are widely expressed but distinct hexokinases dominate specific Cárdenas et al., 1998). Hexokinases phosphorylate a variety of tissues (Katzen and Schimke, 1965, Rogers et al., 1975). HKI is hexose sugars, although their preferred substrate is glucose the most abundant hexokinase in brain and kidney (Griffin (Wilson, 1995). Phosphorylated sugars become substrates for et al., 1992). HKII is the primary hexokinase in muscle and differing metabolic fates, dependent upon the cell type and its adipose tissues (Postic et al., 1994). HKIII predominates in metabolic status (Wilson, 1995, 2003; Cárdenas et al., 1998). A spleen and lymphocytes (Coerver et al., 1998). Glucokinase total of four hexokinases have been identified and extensively (HKIV) is the major hexokinase in glucose sensitive tissues studied in vertebrates, hexokinase I, II, III, and IV (Wilson, such as liver and pancreatic tissues (Printz et al., 1993). In addition to the well-characterized hexokinases, an expressed gene has been identified in mammalian genomes that encode a ⁎ Corresponding author. Department of Laboratory Medicine and Pathobiol- ogy, University of Toronto, 100 College St., Toronto, Ont., Canada M5G 1L5. product that has ADP-dependent glucokinase activity, although Tel.: +1 416 978 0519. this activity has not been demonstrated in vivo (Ronimus and E-mail address: [email protected] (D.M. Irwin). Morgan, 2004), thus its role in glucose metabolism is unclear. 1744-117X/$ - see front matter © 2007 Elsevier Inc. All rights reserved. doi:10.1016/j.cbd.2007.11.002 转载 中国科技论文在线 http://www.paper.edu.cn D.M. Irwin, H. Tan / Comparative Biochemistry and Physiology, Part D 3 (2008) 96–107 97 Mammalian hexokinases I, II, and III are approximately explain the evolution of glucokinase, but also help explain the 100 kDa in size, while glucokinase (HKIV) is only half of this diversification of this gene family and provide insights into how size at only 50 kDa (Wilson, 1995, 2003; Cárdenas et al., 1998). the changes in enzymatic function of these enzymes have Hexokinases from non-vertebrate animals, as well as plants and occurred. fungi are similar in size to glucokinase (Wilson, 1995, 2003; Here we report a characterization of the hexokinase gene Cárdenas et al., 1998). These observations had suggested that family in vertebrates with an analysis of their evolutionary the 100 kDa mammalian hexokinases were the product of a history. Surprisingly we found five, not four, types of duplication of an ancestral 50 kDa hexokinase (Colowick, 1973; hexokinase-like genes. All five of these hexokinase genes Easterby and O'Brien, 1973). Subsequent analysis of the amino were found throughout vertebrates, and in similar genomic acid sequences of hexokinases revealed extensive sequence locations, suggesting that they were products of gene duplica- similarity between the proteins, and clearly demonstrated that tion events that occurred very early in vertebrate evolution. the hexokinase I, II, and III sequences possess duplicated Multiple hexokinase sequences were also found in non- hexokinase domains, and likely were descendent from a gene vertebrate species, including sea urchin and two species of generated by the duplication and fusion of a single hexokinase Ciona, species that are closely related to vertebrates. None of domain-containing ancestor (Griffin et al., 1991; Kogure et al., the non-vertebrate species, though, encoded a hexokinase 1993; Cárdenas et al., 1998). The existence of two hexokinase encoding more than one hexokinase domain. Phylogenetic domains in the HK1, HKII, and HKIII enzymes suggested the analysis of vertebrate and non-vertebrate hexokinases showed possibility that each of these hexokinases may have two active that all the vertebrate sequences were more closely related to sites. Only HKII has been shown to have two active sites (Tsai each other than to any other species, suggesting independent and Wilson, 1996), as the N-terminal hexokinase domains of duplications of the hexokinase genes on multiple lineages, and both HKI and HKIII do not have catalytic activity (White and that the 100 kDa hexokinase originated on the vertebrate lineage Wilson, 1989; Tsai and Wilson, 1997; Tsai, 1999). The after divergence from non-vertebrate species. Our phylogenetic determination of the crystal structure of hexokinase I (Aleshin analysis also showed that the ancestor of all vertebrate et al., 1998a,b) and glucokinase (Kamata et al., 2004) together hexokinases contained two hexokinase domains, thus glucoki- with molecular modeling and site-directed mutagenesis studies nase did lose its N-terminal hexokinase domain. Our phylogeny of glucokinase (Xu et al., 1994, 1995; Mahalingam et al., 1999; also shows that the N-terminal hexokinase domains of HKI and Marotta et al., 2005) have led to a better understanding of the HKIII independently lost hexokinase activity. amino acid residues essential for catalytic activity. While it is clear that HKI, HKII, and HKIII are descendent 2. Materials and methods from a common ancestor that was generated by the duplication of a single hexokinase domain-containing gene, the relationship 2.1. Molecular databases and sequence analysis of glucokinase to these two hexokinase domain-containing hexokinases, as well as the relationships among these Protein databases maintained by the National Center for hexokinases are unclear. Previous sequence analyses have Biotechnology Information (NCBI GenBank, www.ncbi.nlm. yielded support for two different evolutionary histories. A nih.gov) were searched using the BLASTP algorithm (Altschul phylogenetic analysis by Griffin et al. (1991) suggested that the et al., 1997) for sequences similar to human hexokinases I, II, C-terminal hexokinase domains of hexokinases I, II, and III are III, and IV (GCK). Genomic databases of diverse vertebrate more closely related to glucokinase than they were to the N- species (see Table 2) maintained by Ensembl (version 45, www. terminal hexokinase domains of these hexokinases. This ensembl.org) and NCBI were then searched for gene sequences relationship would suggest that glucokinase is descendent similar to human hexokinase genes using the tBLASTN from a hexokinase that contained two hexokinase domains, but algorithm (Altschul et al., 1997). Gene sequences were that it lost its N-terminal hexokinase domain after it diverged downloaded and used for reciprocal BLAST searches to confirm from the other hexokinases (Griffin et al., 1991; Tsai and that they were more similar to human hexokinase genes than to Wilson, 1996). In contrast, Cárdenas et al. (1998) concluded any other gene. Hexokinase sequences from non-vertebrate from their phylogenetic analysis that glucokinase diverged from hexokinase species (i.e., Ciona intestinalis, Ciona savignyi, and the ancestor of the other hexokinase genes prior to the Strongylocentrotus purpuratus) were used to search the duplication of the hexokinase domain (Cárdenas et al.,
Details
-
File Typepdf
-
Upload Time-
-
Content LanguagesEnglish
-
Upload UserAnonymous/Not logged-in
-
File Pages12 Page
-
File Size-