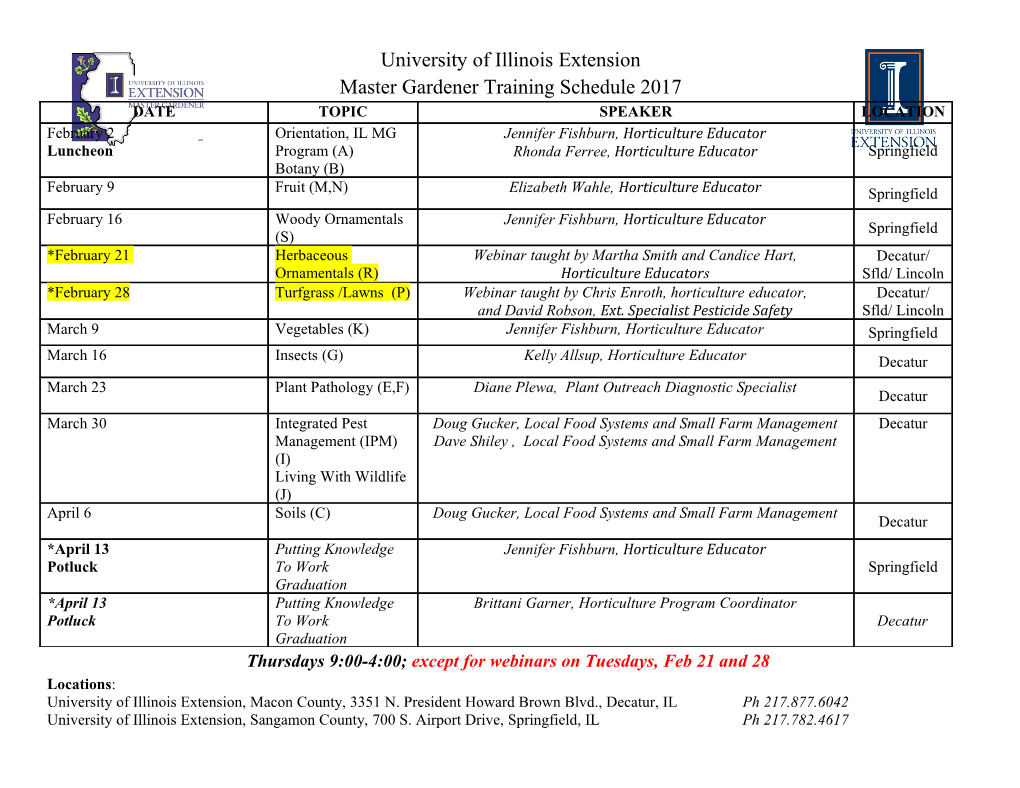
Emmetropization in arthropods: A new vision test in several arthropods suggests visual input may not be necessary to establish correct focusing A thesis submitted to the Graduate School of The University of Cincinnati (Department of Biological Sciences) In partial fulfillment of the requirements for the degree of Master of Science In the Department of Biological Sciences of the College of Arts and Sciences July 2019 By Madeline Owens B.S. Biological Sciences, University of Cincinnati (2016) Committee Chair: Dr. Elke K. Buschbeck, Ph.D. This work will be submitted to The Journal of Experimental Biology Authors: Madeline Owens, Elke K. Buschbeck ABSTRACT The visual systems of vertebrates and invertebrates alike must be able to achieve and maintain emmetropia, a state of correct focusing, in order to effectively execute visually guided behavior. Vertebrate studies, as well as a study in squid, have revealed that for them correct focusing is accomplished through a combination of gene regulation during early development, and homeostatic visual input from the environment that fine-tunes the eye. While eye growth towards emmetropia has been long researched in vertebrates, it is largely unknown how it is established in arthropods. To address this question, we built a micro-ophthalmoscope that allows us to directly measure how a lens projects an image onto the retina in the eyes of small, live arthropods, and to compare the eyes of different light-reared and dark-reared arthropods. Surprisingly, and in sharp contrast to vertebrates, our data on a diverse set of arthropods suggests that visual input in arthropods may not be necessary at least for the initial development of emmetropic eyes. First, we measured the image-forming larval eyes of diving beetles (Thermonectus marmoratus), the eyes of which are known to rapidly and dramatically grow between larval instars. Then we compared jumping spiders (Phidippus audax) after their emergence from their egg case and finally we compared measurements of individual ommatidia of the compound eyes of flesh flies (Sarcophaga bullata) that had developed and emerged either under light or dark conditions. The refractive error of these arthropods was comparable between rearing conditions, suggesting that visual input plays less of a role in developing arthropod eyes than it does in other eyes that develop emmetropia. Although it remains unclear if visual input that is received after the initial development can further improve focusing, these results suggest that in arthropods the initial coordination between the lens refractive power and eye size may be more strongly pre-determined than typically is the case in vertebrates. This research opens new i questions regarding how the growth of arthropod eyes might be regulated and highlights arthropod eyes as a particularly powerful model system for organogenesis. ii Copyright 2019 Madeline Owens iii ACKNOWLEDGEMENTS I would like to thank my advisor, Elke Buschbeck for her guidance, encouragement, and patience. Elke fosters a genuine excitement and passion for learning that will stay with me throughout my life. Throughout my education she has been role model as someone who constantly displays a moral character, work ethic, and general attitude toward unexpected challenges that I strive to emanate in my daily life. I would like to thank my committee members: Annette Stowasser, who spent the time to teach me many of the technical skills that I apply in this paper. Discussing science and working with Annette has been a privilege. Also, Nathan Morehouse, whose enthusiasm for biology and ways to effectively share research inspires me. Thanks to Hailey Tobler for maintaining the labs insect colony with a level of care and attention to detail that few have been able to do. Also, a thank you to Isaiah Giordullo for performing his blind data analysis for this work and to John T Gote and Patrick M. Buttler for collecting jumping spiders in the vicinity of Pittsburgh. A special thanks to Jennifer Hassert, Rose Conley, and Jeremy Didion for always being there for me throughout my graduate experience for support and to talk or brainstorm. Having such wonderful lab peers boosted me up and helped keep me going. Also, a special thanks to Rebecca Meurer in the Biology Department office who helped get me prepared for graduation and constantly goes above and beyond with her willingness to help answer any questions I have. This research was funded by the National Science Foundation under grant IOS1456757 to EKB. iv TABLE OF CONTENTS Introduction – 1 Materials and Methods – 4 Animal rearing Thermonectus marmoratus – 4 Phidippus audax – 5 Sarcophaga bullata – 5 Micro ophthalmoscope measurements – 6 Focal length – 7 Optical assessment-method – 7 Histological assessment – 8 Results – 9 Thermonectus marmoratus – 9 Phidippus audax – 10 Sarcophaga bullata – 12 Discussion – 14 References – 19 Figures – 24 Thermonectus marmoratus – 24 Phidippus audax – 25 Sarcophaga bullata – 26 v LIST OF FIGURES Figure 1) Thermonectus marmoratus optical analysis. (A) External image of T. marmoratus larva in water. Note the principal front facing eyes E1 and E2. (B) Ophthalmoscope image of proximal retina showing two rows of rhabdoms. (C-E) Analysis of E1. (C) Ophthalmoscope measurements showing the object distances (in mm) for the range (represented by bars) over which the E1 photoreceptors are optimally focused for each individual (Dark N=10; Control N=10) as well as their average. (D) Measured focal lengths for dark and control individuals from isolated lenses. (E) Distance between the surface of the retina and the focal plane of the lens of E1 (in μm) based on the measured micro-ophthalmoscope measurements in combination with focal length measurements of each individual. (F-H) The same optical analysis as in C-E but on E2. (F) Object distance for E2 (N=10; Control N=10). (G) Focal length for E2. (H) The distance between the surface of the retina and the focal plane of the lens for E2 based on the focal lengths of the individual’s lenses. Figure 2) Phidippus audax optical analysis. (A) Schematic of the tiered jumping spider retina in principal AM eyes (modified from Stowasser et al., 2017). (B) Ophthalmoscope image of the boomerang shaped AM retina, layer 1. (C) Micro-ophthalmoscope measurements showing the object distances (in mm) including the range for which the photoreceptors of layer 2 are focused for each individual (Dark N=16; Control N=9). The average is indicated as a line. (D) Measurements of focal lengths for AM eye lenses. E) Calculation of the distance between the surface of the retina and the focal plane (and the range of focused images) of the lens for layer 2 based on the micro-ophthalmoscope and focal length measurements for each individual. (F) vi Object distance for layer 1 as established with the micro-ophthalmoscope, (G) Image of Phidippus audax spiderling, illustrating the prominent principal anterior median eyes. (H) The distance between the surface of the retina and the focal plane of the lens for layer 1 based on individual micro-ophthalmoscope and focal lengths measurements. Figure 3) Sarcophaga bullata optical analysis. (A) Ophthalmoscope post-measurement image of the surface of a S. bullata compound eye lens array illustrating bleaching of a single ommatidium (left) which was illuminated by a small square light beam (right). (B) Ophthalmoscope image showing photoreceptors of a single ommatidium. (C) Histological section of S. bullata photoreceptors; scale bar is 50 μm. (D) Ophthalmoscope measurements showing the object distances (in mm) ) for the range (represented by bars) for which the photoreceptors are focused for each individual (Dark N=10; Control daytime N=10; Control nighttime N=10). (E) Measured focal lengths for each individual calculated from the image magnification between the histological and optical images. (Dark N=7; Control daytime N=6; Control nighttime N=9). (F) The calculated distance between the surface of the retina and the focal plane of the lens, based on the micro-ophthalmoscope and lens focal measurements of each individual. vii INTRODUCTION Eyes are among the most complex organs (Land and Nilsson, 2012) and many animals depend on an optimized visual system for survival (Cronin et al., 2014). As such, the visual systems of vertebrates and invertebrates alike must be able to achieve and maintain a state of correct focusing, in order to effectively execute visually guided behavior. Correct focusing relies on the tight coordination between lens refraction and retina position and needs to be established during development and then maintained even if the eye grows. Correctly focused eyes therefore are characterized by a precise match between the focal length of its optics, and the distance between the lens and the retina. Emmetrope eyes are eyes for which images of objects at infinity fall directly onto the retina. If images are positioned in front of the retina eyes are considered to be near-sighted or myopic, and if they are positioned behind the retina, we considered them to be far-sighted, or hyperopic. Typically such deviations are considered to be refractive errors, but note that some invertebrate eyes may have evolved to focus at distances other than infinity (Stowasser et al., 2017) An important question is how eyes develop their optics correctly. This has been investigated intensely in vertebrates (Flitcroft, 2013; McBrien and Barnes, 1984; Troilo, 1992; Wallman and Winawer, 2004; Fledelius et. al., 2014) and has become a particularly important question considering that myopia has been found to be drastically on the rise (Dolgin, 2015). Many arthropods also have sophisticated image-forming eyes (Land and Nilsson 2012) that require precise focusing and therefore likewise must have mechanisms that tightly control eye growth. However, the mechanisms that coordinate lens refraction and the spacing between the lens and retina in developing arthropod eyes remains elusive. Towards gaining a better 1 understanding we here investigate how several arthropod eyes develop with and without access to visual input.
Details
-
File Typepdf
-
Upload Time-
-
Content LanguagesEnglish
-
Upload UserAnonymous/Not logged-in
-
File Pages35 Page
-
File Size-