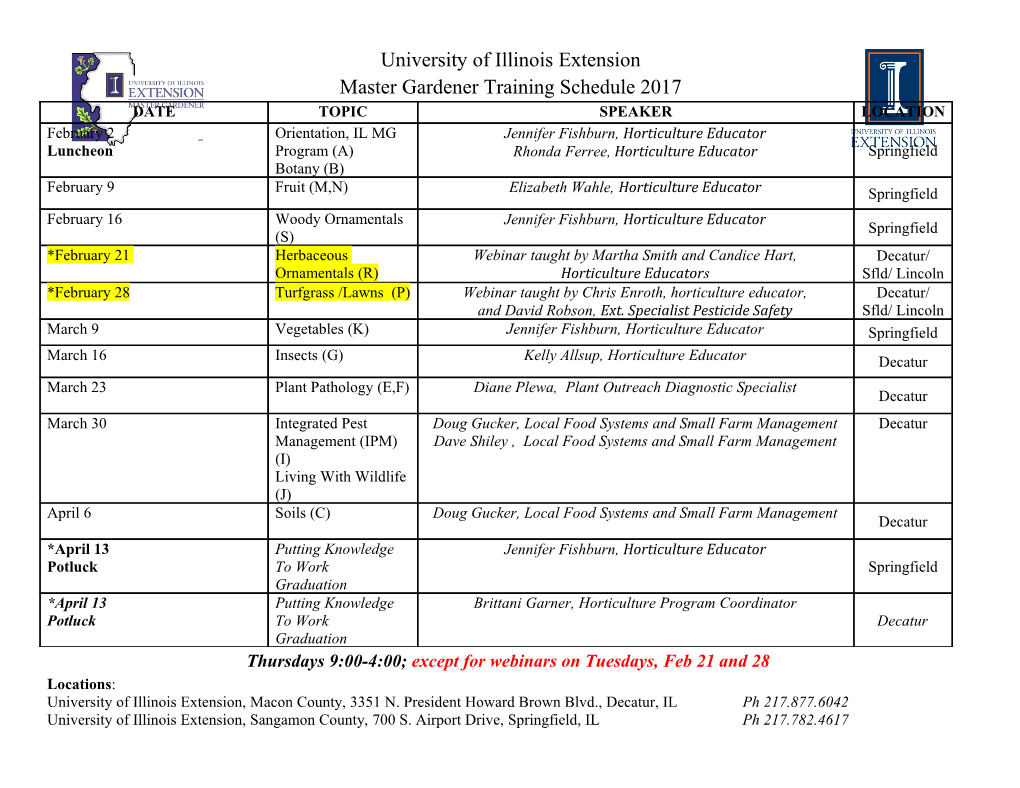
First International Conference on Mars Polar Science 3039.pdf DEEP SPACE 2: THE MARS MICROPROBE PROJECT AND BEYOND. S. E. Smrekar and S. A. Gavit, Mail Stop 183-501, Jet Propulsion Laboratory, California Institute of Technology, 4800 Oak Grove Drive, Pasa- dena CA 91109, USA ([email protected]). Mission Overview: The Mars Microprobe Proj- System Design, Technologies, and Instruments: ect, or Deep Space 2 (DS2), is the second of the New Telecommunications. The DS2 telecom system, Millennium Program planetary missions and is de- which is mounted on the aftbody electronics plate, signed to enable future space science network mis- relays data back to earth via the Mars Global Surveyor sions through flight validation of new technologies. A spacecraft which passes overhead approximately once secondary goal is the collection of meaningful science every 2 hours. The receiver and transmitter operate in data. Two micropenetrators will be deployed to carry the Ultraviolet Frequency Range (UHF) and data is out surface and subsurface science. returned at a rate of 7 Kbits/second. The penetrators are being carried as a piggyback Ultra-low-temperature lithium primary battery. payload on the Mars Polar Lander cruise ring and will One challenging aspect of the microprobe design is be launched in January of 1999. The Microprobe has the thermal environment. The batteries are likely to no active control, attitude determination, or propulsive stay no warmer than -78° C. A lithium-thionyl pri- systems. It is a single stage from separation until mary battery was developed to survive the extreme landing and will passively orient itself due to its aero- temperature, with a 6 to 14 V range and a 3-year shelf dynamic design (Fig. 1). The aeroshell will be made of life. The battery is also designed to withstand a worst a non-erosive heat shield material, Silicon Impreg- case 80,000 g rigid body shock environment. nated Reusable Ceramic Ablator (SIRCA), which has Advanced microcontroller. The microprobes will been developed at Ames Research Center. The aero- include an 8051-based data acquisition and control shell shatters at surface impact, at which time the system with modest data processing capability. This probe separates into an aftbody that remains at the microcontroller is an 8-bit processor with 64K RAM surface and a forebody that penetrates into the sub- and 128K EEPROM. The system is designed for both surface (see Fig. 2). Each probe has a total mass of up very low power (<50 mW at 1 MHz, 1 mW sleep to 3 kg, including the aeroshell. The impact velocity mode) and small volume and mass (<8 cc, 30 to 90 g). will be ~180 m/s. The forebody will experience up to The microcontroller system will also include an inter- 30,000 g’s and penetrate between 0.3 and 2 m, de- nal 12-bit 16-channel analog-to-digital converter pending on the ice content of the soil. The aftbody (ADC). deceleration will be up to 80,000 g. Flexible interconnects for system cabling. One packaging approach for the high shock environment that will be demonstrated is flexible interconnects for system-level cabling. Flex is a Kapton based multi- layer circuit carrier and interconnect technology. The flex circuits will include electrical interconnect layers which are formed with a patented anisotropic bonding material made of thermal glue matrix with embedded solder balls. This bonding technique can withstand temperature extremes and can be used to attach sur- face mount parts using reflow solder. Instruments. The instrument package is designed to demonstrate that valid scientific measurements of both Mars atmospheric conditions and the subsurface Fig. 1. Penetrator stowed within aeroshell. soil characteristics can be obtained using micropene- trators. The primary experiment is the subsurface soil The penetrators arrive in December of 1999. The sampling/water experiment. An auger drill will extend landing ellipse latitude range is 73-77°S. The longi- out from the side of the forebody to approximately 1 tude will be selected by the Mars Surveyor Project to cm (Fig. 2), collecting up to 100 ml of soil. After the place the lander on the polar layered deposits in the sample is collected, the chamber is sealed and the range of 180-230°W. The two micropenetrators are likely to land within 100 km of the Mars Surveyor sample is heated to release water vapor. The vapor Lander, on the polar deposits. The likely arrival date will flow from the sample chamber through a vented is Ls 256, late southern spring. The nominal mission analysis chamber. While in the analysis chamber, the lasts 2 days. A Science Team was selected in April vapor is illuminated by a tunable diode laser (TDL) 1998. and the transmitted power signal is measured to ob- serve the 1.37 µm water absorption line. First International Conference on Mars Polar Science 3039.pdf DS2 AND BEYOND: S. E. Smrekar and S. A. Gavit The forebody also contains an impact accelerome- mass of this type of lander and thus their lower ballis- ter to determine the depth of penetration and presence tic coefficient allow them to reach any latitude on of layering and temperature sensors to estimate soil Mars at any opportunity. They are also capable of conductivity. The single axis accelerometer has a landing at elevations up to 6 km. They are robust with range of -10 to 30,000 g, a precision of 10 g, and a respect to the type of terrain. The current probe is in- sampling rate of 25 kHz. The instrument and expected tended to survive impact into solid ice and penetrate science return are described more fully in [1]. The up to 20 cm. If it hits a rock approximately 10 cm or conductivity of the soil will be estimated using the greater in diameter the probe is likely to fail. How- cooldown rate of the forebody as recorded by two tem- ever, it can survive an incidence angle (the angle be- perature sensors mounted near the outer wall of the tween the trajectory and the surface slope) of up to forebody (Fig. 2). The soil conductivity is primarily a 27°. As the entry trajectory becomes more vertical as function of the grainsize and the presence of frozen it reaches lower elevations, the incidence angle is a volatiles [2]. function of both elevation and local surface slope. Ad- The aft-body houses a surface atmospheric pres- ditionally, the forebody electronics are designed to sure sensor and a descent accelerometer to measure function at -120°C and the battery operates efficiently atmospheric drag on the microprobe. The atmospheric down to -80°C. pressure sensor has a range of 0-25 mbar, a precision The depth of penetration is a function of the di- of 0.01 mbar and an accuracy of 0.03 mbar. The sin- ameter of the forebody, the velocity, and the mass. gle axis descent accelerometer has a range of 1–40 g, Increasing the mass does not severely increase the g- a precision of 25 mg, and a sampling rate of 20 Hz. loads, but for a given diameter is limited by a length Measurement of the atmospheric density can be to width ratio of approximately 5. An additional con- translated into pressure and temperature using the straint is the position of the center of mass relative to hydrostatic equation and the equation of state. the center of pressure. The center of mass must be very far forward in the aeroshell to obtain stability during entry. The size of the aeroshell and overall mass of the system is approximately linearly propor- DS2 PROBE tional to the mass of the penetrator. As a rough ap- DEPLOYED CONFIGURATION proximation, a 20 kg system (aeroshell + probe) might attain a depth of penetration of 2 m in a region with a high ice content. Antenna Changing the instrument package would result in redesign of the electronics. The telecom system would likely have to be redesigned as well, depending on Sun Sensor future relay options. However, packaging techniques Pressure Sensor and Descent for surviving high g loads permit a wide range of in- Transceiver Accelerometer struments. The primary techniques used to withstand Batteries Mars Surface high loads are use of flex interconnect material rather than wire bonds, the use of the smallest possible com- Flex Cable Connection ponents, and either rigid or compliant mounting. The (Data Path) Three-axis mission during could be increased by adding solar Accelerometer Sample Collector power or increasing the battery mass. Drill Motor Water Experiment The possibility for future joint U.S.-French “mi- Instrument Power Electronics Electronics cromissions” to Mars was recently explored in a work- Temperature Microcontroller shop at JPL on May 21–22. Spacecraft of up to 50 kg Sensors can be launched to Mars as piggyback payloads on the French Ariane carrier. These payloads are called ASAPs (Ariane Special Auxiliary Payloads). Many innovative instrument concepts were presented at the Fig. 2. Penetrator in deployed configuration. workshop for looking at oxidants, organics, the depth to the water or ice interface, and mineralogy or geo- Beyond DS2: The technologies developed by DS2 chemistry based on the DS2 design. have much to offer future missions in general and References: . [1] Moersch J. E. and Lorenz R. D., Mars polar missions in particular. Its design objective this volume. [2] Presley M. A. and Christensen P. R. of allowing multiple small landers for network science (1997) JGR-P, 102, 9221-9229. enables multiple measurements of any kind. The low.
Details
-
File Typepdf
-
Upload Time-
-
Content LanguagesEnglish
-
Upload UserAnonymous/Not logged-in
-
File Pages2 Page
-
File Size-