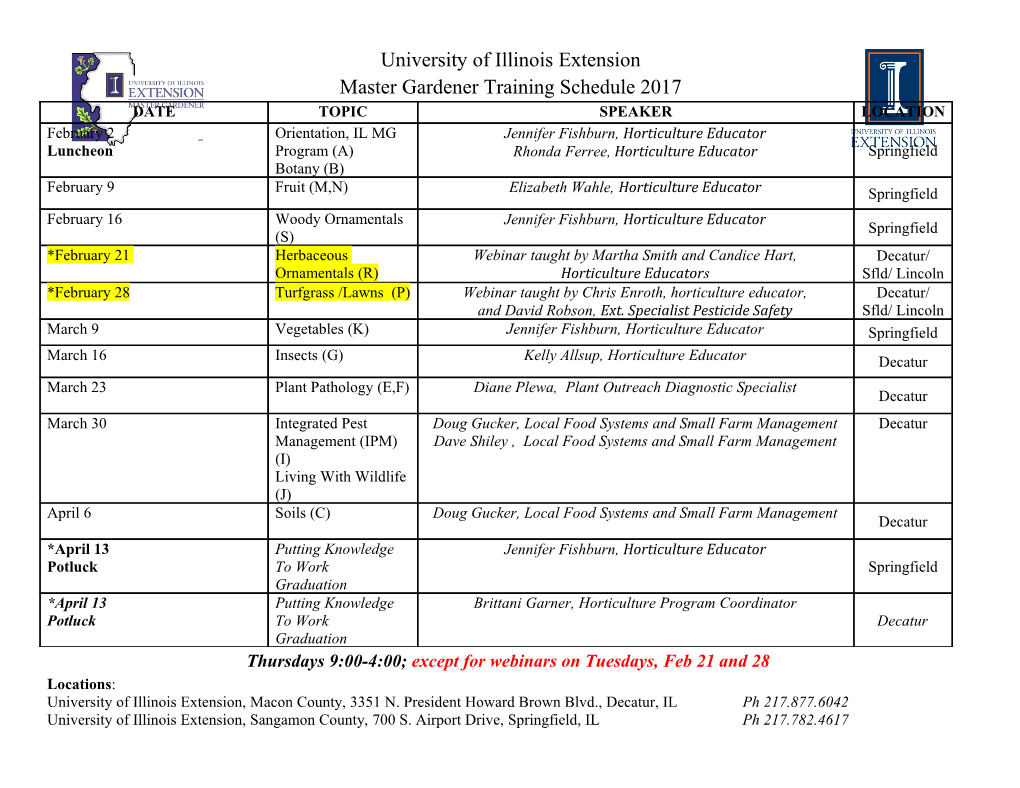
Molecular Dynamics of Folded and Disordered Polypeptides in Comparison with Nuclear Magnetic Resonance Measurement Thesis Presented in Partial Fulfillment of the Requirements for the Degree Master of Science in the Graduate School of The Ohio State University By Lei Yu Graduate Program in Chemistry The Ohio State University 2018 Thesis Committee Rafael Brüschweiler, Advisor Sherwin Singer Copyrighted by Lei Yu 2018 2 Abstract With continuous increase in computer speed, molecular dynamics (MD) simulations have become a crucial biophysical method for the understanding of biochemical processes at atomic detail. However, current molecular mechanics force fields optimized for globular proteins often result in overly collapsed structures for intrinsically disordered proteins (IDPs). In order to study the relevant biological functions of IDPs, it is of great importance to utilize the appropriate force fields. Chemical shifts and J-coupling measurements from solution nuclear magnetic resonance (NMR) experiments serve as a common ground for force field assessment. Various combinations of protein force fields and water models were assessed by the direct comparison between back-calculated NMR parameters and experimental data. TIP4P-D water model was proven to improve the accuracy of back- calculation by producing more realistic dihedral angle ψ distributions. Amber99SBnmr1- ILDN and RSFF2+ force fields were shown to be optimal choices for tripeptide and IDP fragment simulations. The stability of the simulated two-helix bundle (THB) domain in the eukaryotic Na+/Ca2+ exchanger (NCX) suggested the transferable performance of Amber99SBnmr1-ILDN force field in company with TIP4P-D water model in folded proteins. Based on the assessment results, a research plan for future improvement of force fields by rebalancing dihedral angle distributions was proposed. ii Dedication For my family. iii Acknowledgments I would like to express my sincere gratitude towards Prof. Rafael Brüschweiler for his unwavering support throughout my course of study. His multidisciplinary expertise constantly astonishes me while his genuine enthusiasm for science empowers me to pursue further. Besides my advisor, I would like to thank my thesis committee member Prof. Sherwin Singer for his rigorous teaching, insightful comments, and accessible personality. My deep appreciation also goes to Dr. Da-Wei Li for inspiring discussions and prompt guidance. Last but not least, I thank the rest of my group: Dr. Alexandar Hansen, Dr. Lei Bruschweiler-Li, Dr. Bo Zhang, Dr. István Timári, Mouzhe Xie, Jiaqi Yuan, Cheng Wang, Gregory Jameson, Abigail Leggett, Daniel Cantu, and Xinyao Xiang for their assistance and support. iv Vita 2016 ···················· B.S. Chemistry, University of Science and Technology of China 2016 to present ········ Graduate Associate, Department of Chemistry and Biochemistry, The Ohio State University Fields of Study Major Field: Chemistry v Table of Contents Abstract ······························································································· ii Dedication ··························································································· iii Acknowledgments ·················································································· iv Vita ···································································································· v List of Tables ······················································································ viii List of Figures ························································································ x Chapter 1: Introduction ············································································· 1 1.1 Molecular Dynamics (MD) Simulations ·················································· 1 1.2 Nuclear Magnetic Resonance (NMR) Spectroscopy ···································· 3 Chapter 2: Assessment of Molecular Mechanics Force Fields for IDPs by NMR Data ··· 6 2.1 Introduction ··················································································· 6 2.2 Method ························································································· 9 2.2.1 MD simulation ·········································································· 9 2.2.2 Back-calculation of J-coupling constants ·········································· 10 vi 2.2.3 Back-calculation of chemical shifts ················································ 11 2.3 Result and Discussion ····································································· 12 2.3.1 RMSDs of back-calculated J-coupling constants ································· 12 2.3.2 Correlation coefficients of back-calculated J-coupling constants ·············· 14 2.3.3 RMSDs of back-calculated chemical shifts ······································· 16 2.3.4 Comparison with random coil approach ··········································· 19 2.4 Conclusion ·················································································· 20 Chapter 3: MD Simulation of the Two-helix Bundle Domain in the Na+/Ca2+ Exchanger ········································································································ 22 2.1 Introduction ················································································· 22 2.2 Method ······················································································· 25 2.3 Result and Discussion ····································································· 25 2.3.1 Stability of the NMR Structure ······················································ 25 2.3.2 Inter-helical Angles Are Consistent with the Experimental Values ············ 26 2.3.3 Dynamics of the THB Domain ······················································ 29 2.4 Conclusion ·················································································· 30 Chapter 4: Conclusion and Outlook ····························································· 31 Bibliography ························································································ 34 vii List of Tables Table 2.1 RMSD of φ dependent J-coupling constants using different force field and water model combinations. The use of TIP4P-D slightly increased RMSDs for all protein force fields. RSFF2+/TIP3P yielded the smallest RMSD. ·············· 13 Table 2.2 RMSD of ψ dependent J-coupling constants using different force field and water model combinations. Except for CHARMM36m, the RMSDs decreased when TIP4P-D was used with the largest decrease of 12% for Amber99SMnmr1- ILDN. Amer14/TIP4P-D yielded the smallest RMSD. ··························· 13 Table 2.3 φ and ψ equally weighted RMSD of J-coupling constants using different force field and water model combinations. Amber99SBnmr1-ILDN/TIP4P-D yielded the smallest RMSD while RSFF2/TIP4P-D was in the second place. The difference between different force fields was, however, subtle. The best combination was less than 10% better than the worst. ···························· 13 Table 2.4 RMSD of chemical shifts of p53TAD (excluding CO data) and α-Synuclein using different force field and water model combinations. Amber99SBnmr1- ILDN/TIP4P-D yielded the smallest RMSD while RSFF2+/TIP4P-D yielded comparable results. ····································································· 16 Table 3.1 Relative standard deviations of different measures of motion. The relative standard deviations of all 4 measures of motion, namely, the overall RMSD of viii the ordered region of the THB domain, the distance between residue 295 and 310, the distance of residue 295 and 314, and the inter-helical angle, were calculated by dividing the standard deviation by the mean. The relative standard deviation of the overall RMSD is about 2 times larger than the rest, which demonstrates the rigidity of the linker. ·············································· 29 ix List of Figures Figure 2.1 Computational and experimental J-coupling constants. 3J (HN,Hα), 3J (HN,C'), 3J (Hα,C'), 3J (HN,Hβ), 1J (N,Cα), and 2J (N,Cα) coupling constants from available experimental data are plotted against computed ones. Scarlet bars indicate measurement errors. Correlation coefficients, shown on the plots, are low except for 3J (HN,Hα) and 3J (HN,Hβ). The back-calculation overestimated 3J (HN,Hα), 3J (HN,C'), and 2J (N,Cα) coupling constants while underestimated 3J (Hα,C'), 3J (HN,Hβ), and 1J (N,Cα) coupling constants. ······················· 15 Figure 2.2 Computational and experimental chemical shifts in α-Synuclein. Chemical shifts predicted by PPM using 500-nsMD trajectory using Amber99SBnmr1- ILDN/TIP4P-D show overall good correlation with experimental values. The amide proton chemical shifts show weaker correlation due to imperfect force fields and reference error is observed in the plot for N chemical shifts. ······ 18 Figure 2.3 Fraction of RMSD of back-calculated chemical shifts smaller than that of the experimental predictor. When TIP3P water model was used (scarlet), the random coil approach performed better than simulation and PPM combined. When TIP4P-D was used (grey), 12 out of 22 predictions grouped by types of residues and chemical shifts using Amber99SBnmr1-ILDN/TIP4P-D or x RSFF2+/TIP4P-D was better than that using random coil chemical shifts, which indicated both approaches were comparable. ····························· 19 Figure 3.1 Schematic representation of eukaryotic NCX structure (from Jiaqi Yuan, et al., 2018). The updated NCX model consists of a transmembrane domain TM (residues 1-218 and
Details
-
File Typepdf
-
Upload Time-
-
Content LanguagesEnglish
-
Upload UserAnonymous/Not logged-in
-
File Pages52 Page
-
File Size-