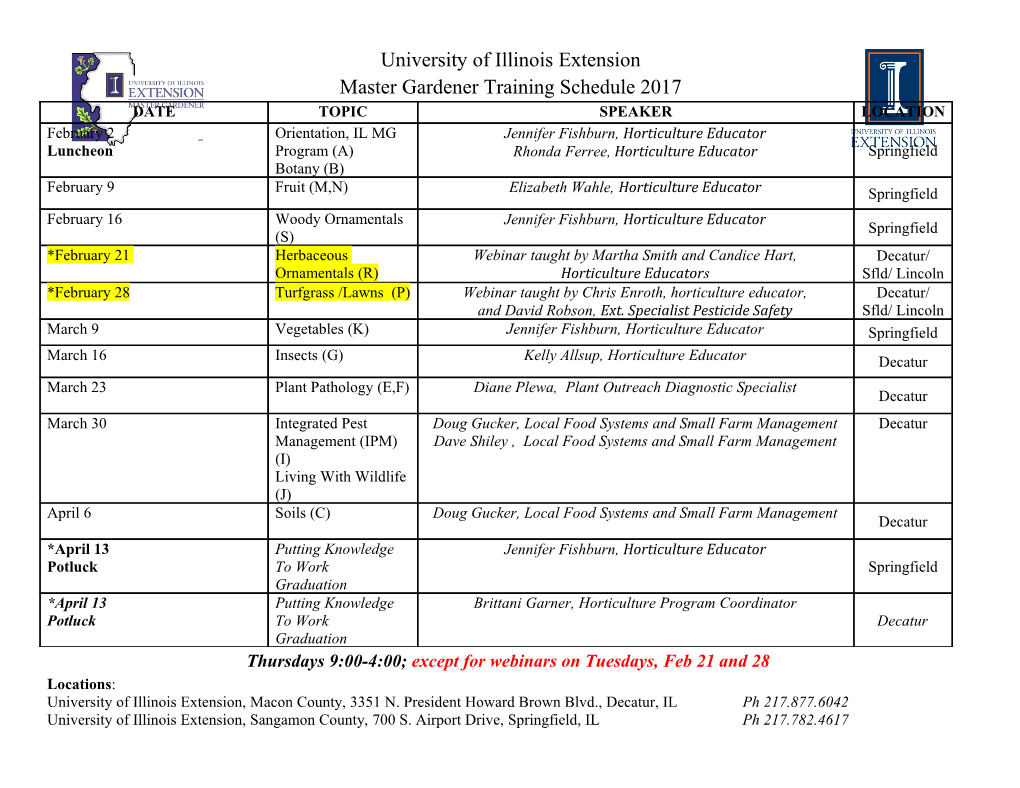
REVIEWS From a quantum-electrodynamical light–matter description to novel spectroscopies Michael Ruggenthaler1*, Nicolas Tancogne-Dejean1*, Johannes Flick1,3*, Heiko Appel1* and Angel Rubio1,2* Abstract | Insights from spectroscopic experiments led to the development of quantum mechanics as the common theoretical framework for describing the physical and chemical properties of atoms, molecules and materials. Later, a full quantum description of charged particles, electromagnetic radiation and special relativity was developed, leading to quantum electrodynamics (QED). This is, to our current understanding, the most complete theory describing photon–matter interactions in correlated many-body systems. In the low-energy regime, simplified models of QED have been developed to describe and analyse spectra over a wide spatiotemporal range as well as physical systems. In this Review, we highlight the interrelations and limitations of such theoretical models, thereby showing that they arise from low-energy simplifications of the full QED formalism, in which antiparticles and the internal structure of the nuclei are neglected. Taking molecular systems as an example, we discuss how the breakdown of some simplifications of low-energy QED challenges our conventional understanding of light–matter interactions. In addition to high-precision atomic measurements and simulations of particle physics problems in solid-state systems, new theoretical features that account for collective QED effects in complex interacting many-particle systems could become a material-based route to further advance our current understanding of light–matter interactions. Spectroscopy (from the Latin speciō ‘look at or view’ and now reaching sub-angstroms and atto seconds. The dif- 1Max Planck Institute for the Greek skopéō ‘to see’) investigates the various properties ferent static and time-resolved spectro scopic techniques Structure and Dynamics of of physical systems over different space, time and energy encompass optical (vibrational, rotational and electronic), Matter and Center for Free- scales by looking at their response to different pertur- magnetic, magnetic-resonance (nuclear and electron Electron Laser Science, bations. The different particles (electrons, protons and spin-resonance), energy-loss, mechanical (atomic force), Hamburg, Germany. 2Center for Computational neutrons) taking part in a physico-chemical process, transport (electronic, spin or heat), and imaging (diffrac- Quantum Physics (CCQ), electro magnetic and thermal radiations or mechanical tion, scanning-tunnelling microscopy and holography) The Flatiron Institute, New deformations can be investigated and simultaneously spectroscopies. Therefore, it is no longer possible to refer York, NY, USA. used as perturbations. In atomic, molecular and solid- to ‘spectroscopy’ without specifying the technique or the 3 Present address: John state physics, spectroscopic methods are specifically spatial and temporal regime. A. Paulson School of Engineering and Applied used to probe and control various aspects of coupled Diverse spectroscopic techniques are applied in differ- 11 Sciences, Harvard University, photon–matter systems, such as the quantum nature of ent fields — from atomic and molecular physics to solid- Cambridge, MA, USA. light1, matter–matter interactions2, electronic3 and nuclear state physics12 and biochemistry13 — and the analysis *e-mail: michael. degrees of freedom4 and collective effects in molecular and and interpretation of the complex experimental data in [email protected]; solid-state systems5. The study of these photon–matter each of these fields are routinely performed by means nicolas.tancogne-dejean@ interactions has informed us, for example, on unknown of purpose-built theoretical tools. These theoretical mpsd.mpg.de; 6,7 [email protected]; states of matter , novel equilibrium and non-equilibrium tools often treat electromagnetic radiation and matter at 8 [email protected]; (driven) topological phases and light-induced super- different levels of approximation, thereby limiting their use [email protected] conductivity9. Over the years, the development and pro- to only particular cases. Usually, photons are considered doi:10.1038/s41570-018-0118 gress of specific experimental spectroscopic techniques only as an external perturbation (often treated classically) Published online 7 Mar 2018 have resulted in remarkable spatial and time resolutions3,10, that probes matter (treated quantum mechanically), NATURE REVIEWS | CHEMISTRY VOLUME 2 | ARTICLE NUMBER 0118 | 1 ©2018 Mac millan Publishers Li mited, part of Spri nger Nature. All ri ghts reserved. REVIEWS whereas the self-consistent back-reaction of matter on equation with the Maxwell equations. The spectrum is photons is neglected. Standard theoretical modelling interpreted as transitions between atomic states, each one can thus be insufficient when the degrees of freedom of interacting or dressed with the classical Maxwell field. photons and matter become equally important. Strong The use of the dressed-electron picture has been known light–matter interaction, as in the case of polariton conden- for a long time, and the coupled Schrödinger–Maxwell sates14, provides a direct example. However, the interaction or Dirac–Maxwell equations form the basic framework between photons and matter, beyond the semi-classical to describe molecular and solid-state spectros copies. description, is also expected to play a role under other However, it is also clear that these equations are simplifi- conditions and could lead, for instance, to the emergence cations of an even more complete theory, which explicitly of collective responses in ensembles of molecules. takes into account the electromagnetic degrees of freedom In this Review, we take a step back from the ever- on a quantized level. Indeed, many experimental results increasing specialization of each of the current theoretical exist that require the simultaneous consideration of the approaches and scrutinize them as different low-energy quantum nature of electrons and the electro magnetic approximations of the general framework of quantum degrees of freedom (see FIG. 1 for a schematic of the evo- electrodynamics (QED). In particular, we focus on lution of our understanding of combined light–matter non-relativistic QED, which is applicable within the typical systems). These include the modification of atomic energy and timescales of molecular and solid-state energy levels in high-Q cavities23, the emergence of quasi- spectros copies (from a few millielectronvolts to a few particles24 such as polaritons, and spectroscopies that thousand electronvolts and from attoseconds to milli- make use of the quantum nature of light22. Traditionally, seconds). A great amount of theoretical and experimental many of these electromagnetic effects (for example, the techniques have been developed to study photon–matter Lamb shift or the finite lifetime of an excited molecular interactions in these energetic and temporal regimes, and state) have been investigated in atomic and small molec- it is beyond the scope of this Review to describe them all or ular systems23,25,26. Although usually small for isolated to provide a comprehensive list of examples; however, we atoms, these electromagnetic effects have an important refer to the relevant literature where possible. We consider role in molecular systems and even more in solid-state mainly optical and electronic spectroscopies, focusing on systems, leading, for example, to the following: the electron–photon phenomena in molecular and solid- screening, polarization and retardation effects observed state systems; we do not explicitly examine direct proton– in light–matter energy transfer induced by attosecond photon interactions but instead describe the nuclei laser pulses27; the emergence of quasi-particles that do (consisting of protons and neutrons) as effective particles. not have a classical counterpart, such as polaritons or Similar considerations can be extended straightforwardly axions28,29; and the formation of novel states of matter, to this additional case. We highlight the limitations of the such as hybrid photon–matter states30,31, exciton–polariton theoretical tools used to describe photon–matter inter- condensates14,32 or light-induced topological states8. Well- actions but also identify new phenomena and hidden known electromagnetic effects in quantum chemistry aspects of these interactions that can be revealed with and solid-state physics33 are long-range intermolecular the development of new tailored spectroscopic tools. interactions and Casimir–Polder forces34,35, Förster reso- 36,37 Polariton When matter and photons are strongly correlated, novel nance energy transfer or the hydrodynamical regime A polariton is a bosonic effects appear, such as changes in the chemical proper- of quantum light38. However, most common theoretical quasi-particle formed by an ties of molecules in optical cavities and the emergence of approaches do not treat these effects self-consistently, excitation, such as an exciton polaritonic chemistry15,16,17,18, strong photon coupling in light- meaning that they do not consider the back-reaction of or plasmon coupled (‘dressed’) 19 with photons. harvesting complexes or attraction between photons matter on the electromagnetic field and vice versa. due to quantum matter20. Such effects have the potential QED provides a general framework to treat electro- Polaritonic chemistry to directly challenge
Details
-
File Typepdf
-
Upload Time-
-
Content LanguagesEnglish
-
Upload UserAnonymous/Not logged-in
-
File Pages16 Page
-
File Size-