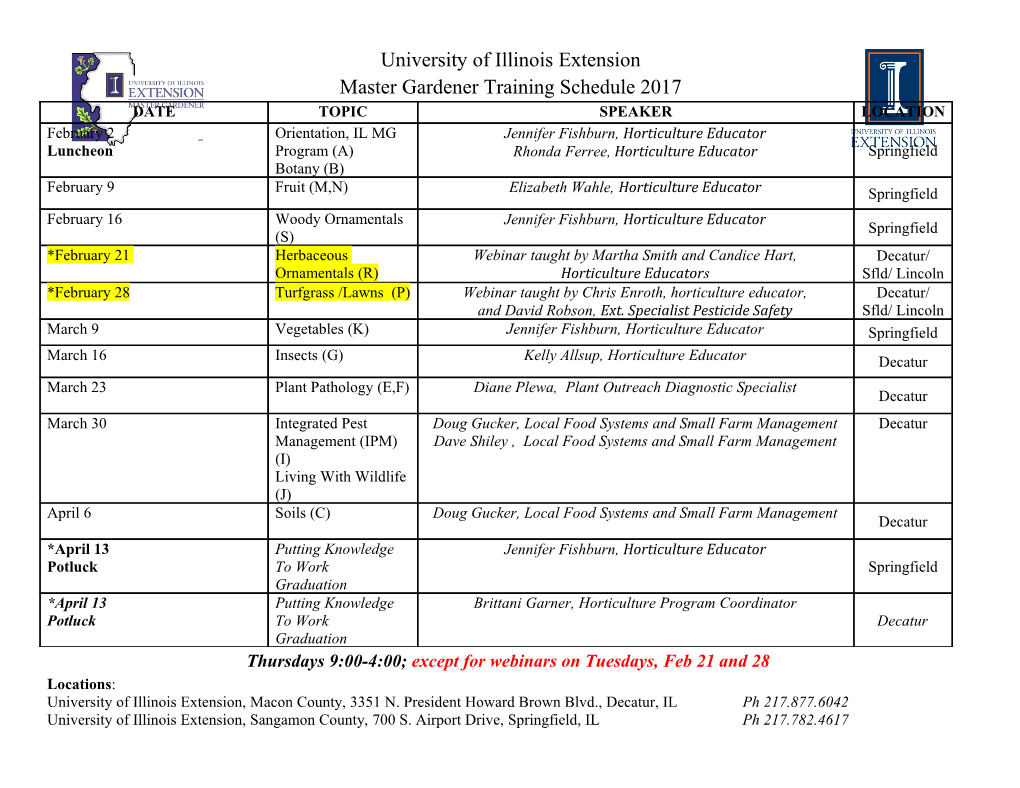
energies Article Spectral Response of Polycrystalline Silicon Photovoltaic Cells under Real-Use Conditions Evaldo C. Gouvêa *, Pedro M. Sobrinho and Teófilo M. Souza Renewable Energy Center, School of Engineering, São Paulo State University (UNESP), Guaratinguetá, Avenida Ariberto Pereira da Cunha 333, Guaratinguetá 12516-410, São Paulo, Brazil; [email protected] (P.M.S.); teofi[email protected] (T.M.S.) * Correspondence: [email protected]; Tel.: +55-12-3123-2777 Received: 11 May 2017; Accepted: 2 August 2017; Published: 10 August 2017 Abstract: The standard test conditions for photovoltaic modules are not capable of reproducing the environmental variations to which the modules are subjected under real operating conditions. The objective of this experimental work is to be an initial study on how the electric energy generation of photovoltaic cells varies according to the different wavelength ranges of the solar light spectrum under real operating conditions. Two modules were installed outdoors; color filters, which allow the passage of light at specific wavelengths, were installed on one of them. The amount of energy produced by the module with the filter was compared to the reference module, and the relative efficiency of each filter was defined. As a result, it was found that crystalline silicon modules do not respond uniformly to sunlight, being more sensitive to the red band (relative efficiency of 23.83%) and less sensitive to the green band (19.15%). The infrared contributes to a significant portion of energy production (relative efficiency of 13.56%), overcoming its negative effect of reducing energy generation capacity due to high photovoltaic cell temperature. Keywords: solar energy; photovoltaic cells; crystalline silicon; solar spectrum; energy efficiency 1. Introduction Most of the modern world uses fossil fuels as its primary source of energy. Coal, oil and natural gas accounted for 66.4% of the world consumption by 2014 [1]. They are abundant, easy to carry and have high energy density; however, they cause great impacts on the environment by the emission of chemical compounds capable of accelerating climate change. Widely available almost everywhere on the globe and practically inexhaustible on a human time scale, solar energy is now a promising energy source with great potential to meet some of the human needs. It can be obtained by the use of photovoltaic cells and modules, whose efficiency depends on their manufacturing process and the environmental conditions of the place where they are installed [2]. It is assumed that all of the radiation received by the solar cells is equivalent to the Air Mass 1.5 (AM1.5) standard. There are daily, seasonal, and regional differences in the spectral distribution of solar radiation at the ground level [3] that could make this assumption imprecise. The AM1.5 standard is used to perform the specification of panels in the laboratory. In addition, laboratory tests are not capable of reproducing the variations of the spectrum, solar radiation, and ambient temperature to which the photovoltaic panels are subjected throughout a day in a real installation. Thus, the panel response under real operating conditions may differ from the technical specifications obtained in tests under controlled conditions. A photovoltaic panel or module (PV) is characterized by parameters that are determined by the manufacturers under the Standard Test Conditions (STC): irradiance of 1000 W·m−2, constant temperature of the cells at 25 ◦C, and the AM1.5 standard sunlight spectrum. However, these parameters cannot be used to estimate the energy output of a module under real operating conditions, Energies 2017, 10, 1178; doi:10.3390/en10081178 www.mdpi.com/journal/energies Energies 2017, 10, 1178 2 of 13 since the energy production in these conditions differs from STC due to variable environmental factors, the geographical location of the module, and the spectral response of each photovoltaic technology [4–6]. Factors that can modify the capacity of the energy production of photovoltaic modules are: the operating temperature, wind speed, irradiance, and the spectrum of sunlight, which have been studied by several authors. According to Ishii, Otani, and Nakashima [7], the operating temperature contributes between 15% and 20% to the reduction of the efficiency of polycrystalline silicon modules. The contribution of the solar spectrum is lower, about 3%. Similarly, Huld and Amillo [8] studied the effect of several variables on the performance of photovoltaic panels. They concluded that variations in the solar spectrum received by crystalline silicon cells leads to a performance variation between −5% and +2%. The influence of temperature and irradiance lead to a performance variation between −15% and +5%. The assembly type affects the panel temperature: non-ventilated assemblies such as the ones made on rooftops result in an increase in the panel temperature. This increase can be minimized if there is wind incidence, which reduces the temperature difference between the module and the environment, and if the operating regime is the maximum power point tracking [6,9]. In addition, the temperature difference between the panel and the environment depends on efficiency: the higher the efficiency of the module, the lower the losses and working temperature [6]. There are several active and passive cooling methods for photovoltaic panels, in order to increase efficiency: heat exchangers with aluminum or copper pipes run by refrigerant fluid; heatsinks with fin coolers, with natural or artificial ventilation; continuous spraying of water on the surface of the panels; and direct liquid circulation in the rear of modules with solar concentration [10–12]. The maximum power that a photovoltaic panel can reach varies according to the variations of the solar spectrum received by the component and the angle incidence of light, which change throughout the day and year [4,8]. However, the STC do not consider these variations when determining the nominal power of the modules, but other authors have recently addressed this topic in their studies. Cañete, Carretero, and Sidrach-de-Cardona [4] compared the efficiency of four photovoltaic panel technologies under real climatic conditions in southern Spain and concluded that thin-film cells show greater variation in energy production according to the seasons, being consistent with [8]. In addition, they reported that the daily efficiency of polycrystalline silicon cells was shown to be 7.6% lower under real operating conditions than in the standard test conditions due to the working high temperature. The energy production of photovoltaic systems can be estimated by the atmospheric conditions of the installation site. Different combinations of irradiance and temperature are considered in several works [13–15], but variations of the spectrum are generally disregarded. The influence of spectral variations on the PV performance depends on the spectral response of each photovoltaic cell technology [5]. In addition, the effective spectral irradiance—that is, the one that actually contributes to the current generation in the panel—is different from the standard AM1.5 spectrum used in laboratory tests of cells and modules, which covers a wide range of wavelengths. In general, solar panels do not respond to the full AM1.5 spectrum [5,16–18]. The impact of the spectral distribution on energy production varies for each month of the year and decreases as the latitude of the installation site of the photovoltaic modules decreases [19]. In addition, the spectral impact can be reduced by using a wavelength range between 350 nm and 1050 nm for analysis [20]. In the same way that cooling methods were projected to reduce the impact of temperature and increase the efficiency of photovoltaic systems, several research works have been carried out to find ways to increase energy production by modifying the light spectrum received by the panel. Some of these methods include: optical traps [21], antireflective layers [22–25], luminescent down-shifting layers [26–29] and color filters [30,31]. Landrock et al. [31] developed a solar simulator with tungsten lamps and color correction using low-cost filters. The light source was evaluated according to three parameters: spectral response, spatial uniformity, and temporal stability. The spectrum of the lamps Energies 2017, 10, 1178 3 of 13 was verified with a high-precision spectrometer and was corrected with the installation of four color filters. In the aforementioned works, photovoltaic cells were always exposed to the complete sunlight spectrum, in both controlled laboratory and real-use conditions. The influence of the spectrum is obtained through the use of spectrometers and sophisticated mathematical methods (i.e., by indirect methods). In this work, photovoltaic cells are exposed to just a specific wavelength range of the solar spectrum at a time through the use of color filters. In this way, it is possible to directly verify the effect of each wavelength range of sunlight on the capacity of the energy production of photovoltaic modules, without using complex mathematical methods or expensive measurement equipment like a spectrometer. Furthermore, PV cells are exposed to real variations of environmental temperature, solar spectrum, and the angle of incidence of light—aspects that solar simulators cannot reproduce in the laboratory. The objective of this experimental work is to be an initial study on how the electric energy generation of polycrystalline silicon photovoltaic
Details
-
File Typepdf
-
Upload Time-
-
Content LanguagesEnglish
-
Upload UserAnonymous/Not logged-in
-
File Pages13 Page
-
File Size-