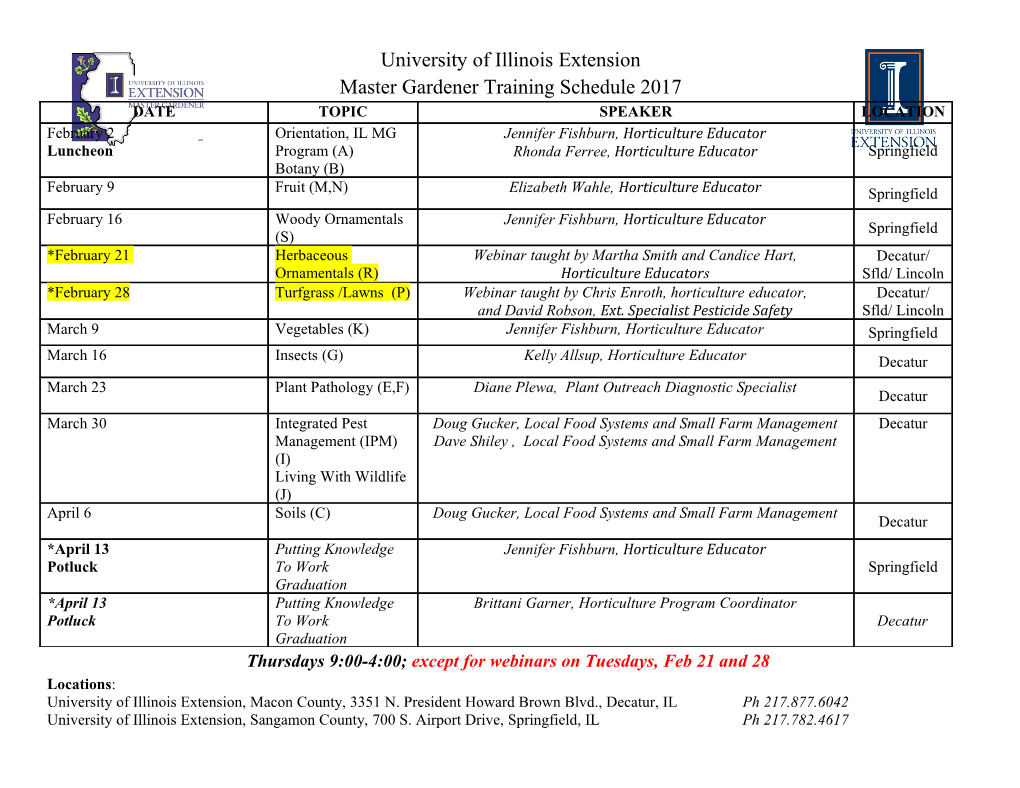
International Journal of Molecular Sciences Article Effect of A22 on the Conformation of Bacterial Actin MreB Elvis Awuni 1 and Yuguang Mu 2,* 1 Department of Biochemistry, School of Biological Sciences, CANS, University of Cape Coast, Cape Coast 00233, Ghana; [email protected] 2 School of Biological Sciences, Nanyang Technological University, 60 Nanyang Drive, Singapore 637551, Singapore * Correspondence: [email protected]; Tel.: +65-63162885 Received: 23 January 2019; Accepted: 25 February 2019; Published: 15 March 2019 Abstract: The mechanism of the antibiotic molecule A22 is yet to be clearly understood. In a previous study, we carried out molecular dynamics simulations of a monomer of the bacterial actin-like MreB in complex with different nucleotides and A22, and suggested that A22 impedes the release of Pi from the active site of MreB after the hydrolysis of ATP, resulting in filament instability. On the basis of the suggestion that Pi release occurs on a similar timescale to polymerization and that polymerization can occur in the absence of nucleotides, we sought in this study to investigate a hypothesis that A22 impedes the conformational change in MreB that is required for polymerization through molecular dynamics simulations of the MreB protofilament in the apo, ATP+, and ATP-A22+ states. We suggest that A22 inhibits MreB in part by antagonizing the ATP-induced structural changes required for polymerization. Our data give further insight into the polymerization/depolymerization dynamics of MreB and the mechanism of A22. Keywords: molecular dynamics; simulations; actin-like MreB; conformational change; polymerization; depolymerization 1. Introduction The bacterial actin-like MreB forms the cytoskeleton network, and is involved in several life processes of rod-shaped bacteria [1–10]. MreB polymerizes into filaments which are made of two straight and antiparallel strands, unlike the two twisted and parallel strands of eukaryotic actin [11]. Figure1a–c shows the crystal structures of the monomeric (PDB ID: 4CZK [ 11]), single protofilament (PDB ID: 4CZI [11]), and double protofilament (PDB ID: 4CZJ [11]), respectively, of Caulobacter (C) cresentus MreB (CcMreB). The four subdomains (IA, IB, IIA, and IIB) of MreB, A22, and ATP binding sites are indicated in Figure1a. To form a single filament, adjacent monomeric chains of MreB interact longitudinally at the intraprotofilament interfaces (Figure1b). Opposite chains interact laterally at the interprotofilament interfaces to form a double protofilament as illustrated in Figure1c. Thus, the polymerization of MreB involves both single filament and double filament formation. The antibiotic molecule A22 (Figure1d) has been shown to affect bacteria by targeting MreB [8,12,13], but its mechanism is yet to be clearly and fully understood. In a previous study [14], we carried out molecular dynamics (MD) simulations of monomeric bacterial actin-like MreB in complex with different nucleotides (NTs) and A22, and suggested that A22 impedes the release of Pi from the active site of MreB after the hydrolysis of ATP thus resulting in filament instability. On the basis of the observations we made [14], the fact that Pi release occurs on a similar timescale to polymerization [15–17], and that polymerization can occur in the absence of NTs [18], we proposed a hypothesis that A22 interferes with the conformational change in MreB that is required Int. J. Mol. Sci. 2019, 20, 1304; doi:10.3390/ijms20061304 www.mdpi.com/journal/ijms Int. J. Mol. Sci. 2019, 20, 1304 2 of 14 Int. J. Mol. Sci. 2019, 20, x FOR PEER REVIEW 2 of 14 for polymerization. In this study, MD simulations of the MreB protofilament in the empty (apo), wereATP+, carried and ATP-A22+out to test statesthis hypothesis. were carried We obse out torved test that this (i) hypothesis. ATP induces We a conformational observed that change (i) ATP ininduces MreB athat conformational could favor changethe formation in MreB of that stable could single favor and the formationdouble protofilaments, of stable single and and (ii) double A22 interferesprotofilaments, with the and generation (ii) A22 interferes of this favorable with the generation conformation of this and favorable induces conformationa structure that and may induces not supporta structure polymerization that may not of support MreB into polymerization stable filaments. of MreB into stable filaments. Figure 1. Forms of MreB and structure of A22. (a) Monomeric structure of MreB. Subdomains IA, IB, Figure 1. Forms of MreB and structure of A22. (a) Monomeric structure of MreB. Subdomains IA, IB, IIA, and IIB as well as the ATP and A22 binding sites are indicated. The α-helix and β-sheet secondary IIA, and IIB as well as the ATP and A22 binding sites are indicated. The α-helix and β-sheet secondary structural elements are labeled. (b) Structure of a single protofilament of MreB composing of chains A, structural elements are labeled. (b) Structure of a single protofilament of MreB composing of chains B, and C. The intraprotofilament interfaces are indicated. (c) Structure of double protofilament of MreB. A, B, and C. The intraprotofilament interfaces are indicated. (c) Structure of double protofilament of The antiparallel strands and the interprotofilament interface are shown. (d) Structure of A22. MreB. The antiparallel strands and the interprotofilament interface are shown. (d) Structure of A22. 2. Results and Discussion 2. Results and Discussion 2.1. A22 Impedes ATP-Induced Backbone Conformational Change in MreB 2.1. A22To Impedes determine ATP-Induced any conformational Backbone Conformational change in the Change apo, ATP+, in MreB and ATP-A22+ MreB, root mean squareTo deviationdetermine (RMSD)any conformational analysis was change carried in out the on apo, the ATP+, backbone and atomsATP-A22+ of chain MreB, B inroot all mean three squaresimulations deviation of each (RMSD) state. Theanalysis RMSDs was were carried calculated out on by the using backbone the corresponding atoms of chain equilibrated B in all initialthree simulationsstructure of of each each state state. of MreBThe RMSDs as the reference.were calculat Theed RMSD by using values the of corresponding the last 50 ns simulationsequilibrated ofinitial each structurestate were of usedeach state to generate of MreB RMSD as the distributionreference. The curves. RMSD The values results, of the as last reported 50 ns simulations in Figure2, of reveal each statethat therewere isused a relatively to generate large RMSD conformational distribution change curves. in The the results, ATP+ state as reported (broad redin Figure distribution 2, reveal curves) that thereof MreB. is a relatively In the apo large and ATP-A22+conformational forms change (narrow in cyanthe ATP+ and greenstate (broad distribution red distribution curves, respectively), curves) of MreB.however, In the the apo backbone and ATP-A22+ atoms undergo forms relatively (narrow smallcyan conformationaland green distribution changes curves, as compared respectively), with the however,ATP+ form. the The backbone closeness atoms of the undergo backbone relatively atom distributions small conformational of the apo changes and ATP-A22+ as compared MreB formswith thesuggests ATP+ thatform. A22 The impedes closeness ATP-induced of the backbone conformational atom distributions change. of the apo and ATP-A22+ MreB forms suggests that A22 impedes ATP-induced conformational change. Int. J. Mol. Sci. 2019, 20, 1304 3 of 14 Int. J. Mol. Sci. 2019, 20, x FOR PEER REVIEW 3 of 14 Figure 2. Figure 2. Backbone-atom root mean square deviation (RMSD) distribution curves of apo, ATP+,ATP+, and ATP-A22+ MreB. The solid, dashed, and dotted cyan lines represent backbone RMSD distributions of ATP-A22+ MreB. The solid, dashed, and dotted cyan lines represent backbone RMSD distributions of chain B from simulations 1, 2, and 3, respectively, of the apo filament. The solid, dashed, and dotted red chain B from simulations 1, 2, and 3, respectively, of the apo filament. The solid, dashed, and dotted lines represent backbone RMSD distributions of chain B from simulations 1, 2, and 3, respectively, of red lines represent backbone RMSD distributions of chain B from simulations 1, 2, and 3, respectively, the ATP+ filament. The solid, dashed, and dotted green lines represent backbone RMSD distributions of the ATP+ filament. The solid, dashed, and dotted green lines represent backbone RMSD of chain B from simulations 1, 2, and 3, respectively, of the ATP-A22+ filament. distributions of chain B from simulations 1, 2, and 3, respectively, of the ATP-A22+ filament. 2.2. MreB Adopts One Main Low-Energy Structure in the Apo, ATP+, and ATP-A22+ States 2.2. MreB Adopts One Main Low-Energy Structure in the Apo, ATP+, and ATP-A22+ States To visualize the most essential dynamics and structural variations in the apo, ATP+, and ATP-A22+ statesTo of visualize MreB, principal the most component essential dynamics analyses (PCA) and st wereructural performed variations on thein the backbone apo, ATP+, atoms and of chainATP- BA22+ of each states state of MreB, using principal the last 50component ns trajectories analyses of the(PCA) simulations. were performed The gmxcovar on the backbone tool in Gromacsatoms of 5.4.1chain was B of usedeach tostate generate using the eigenvectors. last 50 ns trajectori For thees three of the simulations simulations. of eachThe gmxcovar MreB state, tool the in trajectory Gromacs with5.4.1 thewas lowest used to cosine generate content eigenvec wastors. selected For forthe thethree generation simulations of aof 2D each projection MreB state, and the a free trajectory energy landscapewith the lowest (FEL) cosine plot using content gmxanaeig was selected and gmxfor the sham generation utilities inof Gromacs,a 2D projection respectively. and a free An energy earlier studylandscape suggested (FEL) thatplot ausing lower gmxanaeig cosine content and valuegmx sham (preferably, utilities <0.2 in Gromacs, on the scale respectively. of 0 to 1) is An indicative earlier ofstudy efficient suggested sampling that fora lower FEL analysiscosine content [19]. value (preferably, <0.2 on the scale of 0 to 1) is indicative of efficientIn each sampling case, the for first FEL and analysis the second [19].
Details
-
File Typepdf
-
Upload Time-
-
Content LanguagesEnglish
-
Upload UserAnonymous/Not logged-in
-
File Pages14 Page
-
File Size-