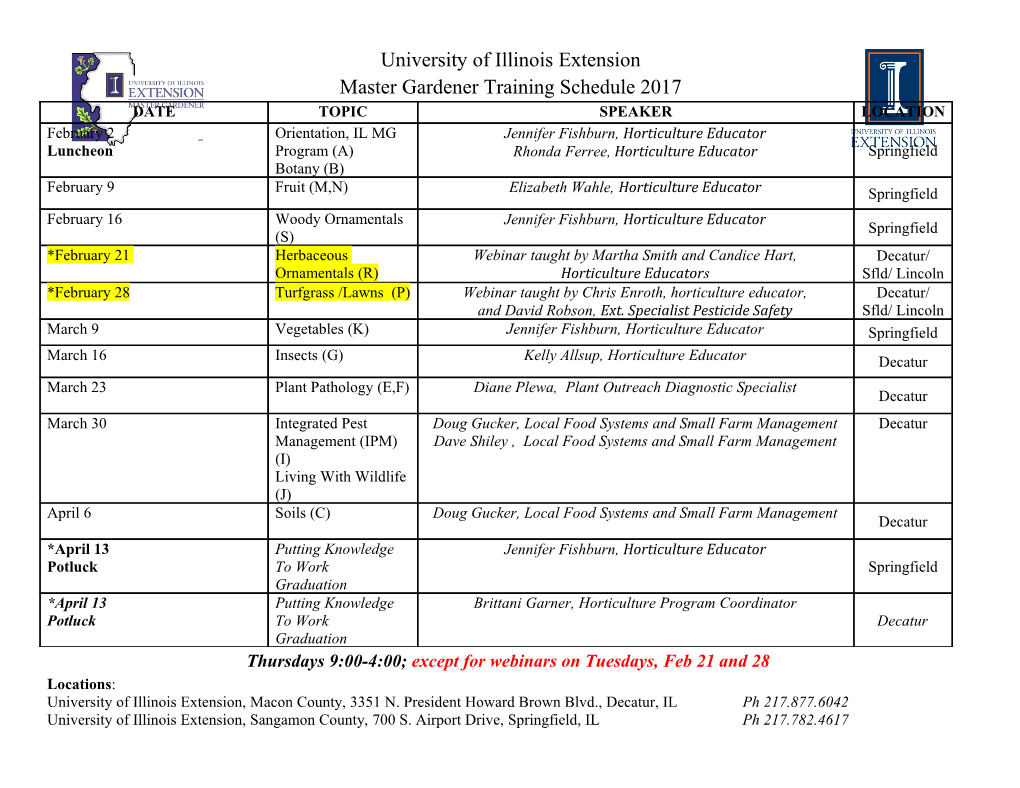
Artificial miRNAs mitigate shRNA-mediated toxicity in the brain: Implications for the therapeutic development of RNAi Jodi L. McBride*, Ryan L. Boudreau*, Scott Q. Harper*†, Patrick D. Staber*, Alex Mas Monteys*, Ineˆ s Martins*, Brian L. Gilmore*, Haim Burstein‡, Richard W. Peluso‡, Barry Polisky§, Barrie J. Carter‡, and Beverly L. Davidson*¶ʈ** Departments of *Internal Medicine, ¶Molecular Physiology and Biophysics, and ʈNeurology, University of Iowa, Iowa City, IA 52242; ‡Targeted Genetics, 1100 Olive Way, Suite 100, Seattle, WA 98101; and §Sirna Therapeutics, 1700 Owens Street, San Francisco, CA 94158 Communicated by David E. Housman, Massachusetts Institute of Technology, Cambridge, MA, February 27, 2008 (received for review February 10, 2008) Huntington’s disease (HD) is a fatal, dominant neurodegenerative in the setting of two normal mouse HD homolog (HDh) alleles. disease caused by a polyglutamine repeat expansion in exon 1 of Although allele-specific targeting of disease transcripts for HD the HD gene, which encodes the huntingtin protein. We and others therapy would be ideal, to date no prevalent SNP residing on the have shown that RNAi is a candidate therapy for HD because mutant transcript has been identified. Therefore, we undertook expression of inhibitory RNAs targeting mutant human HD trans- studies to identify inhibitory RNAs that would target both mouse genes improved neuropathology and behavioral deficits in HD HDh and human HD transcripts, with the intention of testing the mouse models. Here, we developed shRNAs targeting conserved efficacy of reducing the expression of both alleles in a knockin sequences in human HD and mouse HD homolog (HDh) mRNAs to model of HD (10). Here we describe the surprising finding of initiate preclinical testing in a knockin mouse model of HD. We neurotoxicity in mouse brain caused by some, but not all, shRNA screened 35 shRNAs in vitro and subsequently narrowed our focus expression vectors screened in vivo and the notable reduction in to three candidates for in vivo testing. Unexpectedly, two active toxicity after moving those toxic inhibitory RNAs into miRNA- shRNAs induced significant neurotoxicity in mouse striatum, al- based delivery systems. though HDh mRNA expression was reduced to similar levels by all three. Additionally, a control shRNA containing mismatches also Results induced toxicity, although it did not reduce HDh mRNA expression. shRNAs Cause Striatal Toxicity in Mice. We first designed and Interestingly, the toxic shRNAs generated higher antisense RNA screened shRNAs (driven by the mouse U6 promoter) that target levels, compared with the nontoxic shRNA. These results demon- conserved sequences spanning human HD and mouse HDh strate that the robust levels of antisense RNAs emerging from mRNAs [Fig. 1A and supporting information (SI) Table S1], shRNA expression systems can be problematic in the mouse brain. taking into consideration the most recent siRNA design rules Importantly, when sequences that were toxic in the context of (11–13). Silencing of HD mRNA measured by quantitative shRNAs were placed into artificial microRNA (miRNA) expression real-time PCR (QPCR) and dot blot analysis revealed a decrease systems, molecular and neuropathological readouts of neurotox- in huntingtin protein expression after transfection of shRNA icity were significantly attenuated without compromising mouse expression plasmids into mouse C2C12 and human-derived HEK HDh silencing efficacy. Thus, miRNA-based approaches may pro- 293 cell lines (data not shown). Of the 35 shRNAs tested, three vide more appropriate biological tools for expressing inhibitory were chosen for further study based on silencing efficacy. The RNAs in the brain, the implications of which are crucial to the shRNAs target sequences in exons 2, 8, and 30 of HD mRNAs development of RNAi for both basic biological and therapeutic and are henceforth referred to as sh2.4, sh8.2, and sh30.1, applications. respectively (Fig. 1B). Western blot analysis demonstrated that these shRNAs, but not mismatch (mis) control shRNAs, reduce gene therapy ͉ Huntington’s disease ͉ RNAi ͉ AAV endogenous huntingtin protein expression in mouse C2C12 cells (Fig. 1C). Similar results were seen in human-derived HEK 293 he ability of siRNAs to silence target genes was first demon- cells. Tstrated in 1998 by Andrew Fire et al. (1) and has since emerged To examine the long-term effects of brain-delivered shRNAs in as a revolutionary strategy to reduce target gene expression. RNAi the CAG140 knockin mouse model of HD (10), U6-shRNA occurs naturally in cells as a posttranscriptional regulatory mech- expression cassettes were cloned into adeno-associated viral vectors anism mediated by endogenous miRNAs (2–5). RNAi is hypoth- (AAV serotype 2/1) (Fig. 2A). AAVs also contained a humanized esized to have evolved as a cellular coping mechanism providing the Renilla GFP (hrGFP) expression cassette to identify the distribu- cell a means to decrease the expression of various deleterious tion and types of cells transduced. Five-week-old CAG140 knockin viruses and transposons (6, 7). In recent years, scientists have mice were injected bilaterally into the striatum with AAVsh2.4- coopted this biological process to reduce the expression of target GFP, AAVsh8.2-GFP, AAVsh30.1-GFP, or AAV-GFP (viral con- mRNAs by using exogenously applied siRNAs, shRNAs, or artifi- trol) and killed 15 weeks later. Robust expression of GFP was cial miRNAs. Aside from the widespread basic biological applica- tions of RNAi, the ability to reduce gene expression marks a major Author contributions: J.L.M., R.L.B., and S.Q.H. contributed equally to this work; J.L.M., advance toward the development of disease therapies, particularly R.L.B., S.Q.H., B.P., B.J.C., and B.L.D. designed research; J.L.M., R.L.B., S.Q.H., P.D.S., A.M.M., for dominantly inherited disorders. I.M., B.L.G., H.B., and R.W.P. performed research; J.L.M., R.L.B., S.Q.H., and B.L.D. analyzed Among the dominant diseases that may benefit from RNAi- data; and J.L.M., R.L.B., S.Q.H., and B.L.D. wrote the paper. based therapies is Huntington’s disease (HD). Our laboratory (8) Conflict of interest statement: B.L.D. was a consultant for Sirna Therapeutics, Inc.. and others (9) have previously demonstrated that partial reduction †Present address: Center for Gene Therapy, Department of Pediatrics, Ohio State University, of mutant huntingtin expression by viral delivery of shRNAs is Columbus, OH 43205. efficacious in preventing the development of motor deficits and **To whom correspondence should be addressed. E-mail: [email protected]. neuropathology in transgenic mouse models of HD. In these This article contains supporting information online at www.pnas.org/cgi/content/full/ proof-of-principal studies, the therapeutic effect on disease pheno- 0801775105/DCSupplemental. type was studied by knocking down a mutant human HD transgene © 2008 by The National Academy of Sciences of the USA 5868–5873 ͉ PNAS ͉ April 15, 2008 ͉ vol. 105 ͉ no. 15 www.pnas.org͞cgi͞doi͞10.1073͞pnas.0801775105 Downloaded by guest on September 27, 2021 AAVshRNA-GFP A A ITR ITR 1 2 8 30 U6shRNA TTTT CMV hrGFP polyA B sh2.4 B Anterior U U 5‘ C C GACCGUGUGAAUCAUUGUCUA C CUGGCACACUUAGUAACAGAU U 3‘-UU A G C U striatum striatum striatum striatum sh8.2 U U 5‘ C C CAGCUUGUCCAGGUUUAUGAA C GUCGAACAGGUCCAAAUACUU U 3‘-UU A G striatumglobus pallidus globus pallidus globus pallidus C U C 1.0 sh30.1 U U 5‘ C C GGAUACCUGAAAUCCUGCUUU C 0.8 CCUAUGGACUUUAGGACGAAA U 3‘-UU A G C U 0.6 * * * C 0.4 0.2 Relative HDh mRNA Untreatedsh8.2 sh2.4mis sh30.1 mis sh8.2 missh2.4sh30.1shLacZ 0 huntingtin sh2.4 sh8.2 sh30.1 GFP beta-catenin D GFP sh2.4 sh8.2 sh30.1 Fig. 1. In vitro screening of shRNAs targeting human HD and mouse HDh transcripts. (A) Thirty-five shRNAs (bars above cartoon) targeting conserved sequences (Table S1) spanning human HD and mouse HDh mRNAs were generated with consideration for sequences that promote proper loading of AAVsh8.2-GFP the antisense strands into the RISC. Plasmids expressing U6-driven shRNAs DARPP-32 were transfected into HEK 293 cells, and HD gene silencing was evaluated by QPCR and protein dot blot analyses 48 h after transfection. (B) Three candidate shRNAs targeting sequences in exons 2 (sh2.4), 8 (sh8.2), and 30 (sh30.1) were Iba1 chosen for further study (red bars above cartoon in A). (C) shRNA expression plasmids were transfected into mouse C2C12 cells, and endogenous hunting- Fig. 2. HD shRNAs cause sequence-specific striatal toxicity in mice. (A) tin protein levels were evaluated by Western blot analyses 48 h after trans- Diagram of the recombinant AAV2/1 viral vectors containing shRNA and fection. Mismatch (mis) controls contain 4-bp changes that render the shRNAs hrGFP expression cassettes. (B) Photomicrographs represent the rostral-to- ineffective. -Catenin serves as the loading control. caudal distribution of hrGFP-positive cells in mouse brain after direct injection of virus into the striatum. (Scale bar: 500 m.) (C) QPCR analysis measuring HDh mRNA levels in shRNA-treated mouse striata demonstrates similar silenc- observed in cells throughout the rostral/caudal extent of the stria- ing efficacies among sh2.4, sh8.2, and sh30.1. Mice were injected into the tum and within fibers of the globus pallidus (Fig. 2B). Immuno- striatum with AAVsh2.4-GFP, AAVsh8.2-GFP, AAVsh30.1-GFP, or AAV-GFP, fluorescence analyses indicated that GFP-positive cells colocalized and RNA was harvested 4 months later from GFP-positive striata. All values with a neuronal marker (NeuN), but not with markers for astrocytes were normalized to -actin and are shown relative to AAV-GFP-treated brains.
Details
-
File Typepdf
-
Upload Time-
-
Content LanguagesEnglish
-
Upload UserAnonymous/Not logged-in
-
File Pages6 Page
-
File Size-