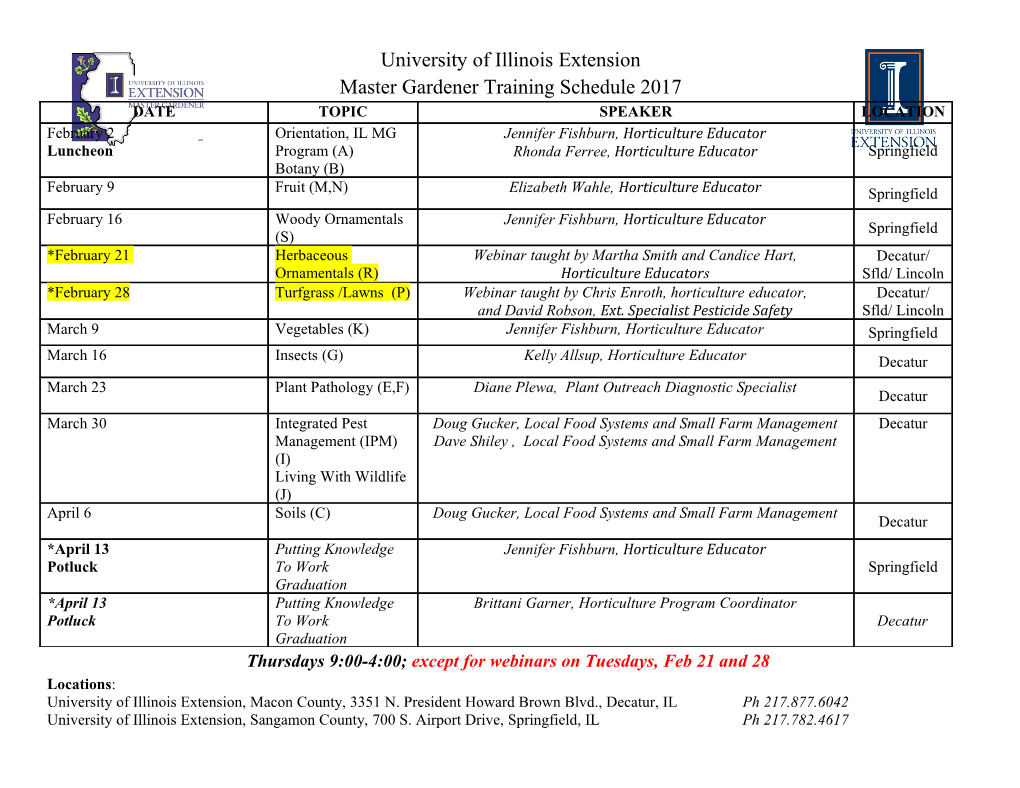
Introduction to D-modules in representation theory Pavle Pandˇzi´c University of Zagreb and University of Freiburg 35th Winter School \Geometry and Physics" Srn´ı,January 2015. LECTURE I To a complex semisimple Lie algebra g, one can attach its flag variety B. B is a smooth projective algebraic variety. To study g-modules, one can instead study certain related D-modules on B. D-modules are sheaves of modules over the sheaves of differential operators. Goal B is a smooth projective algebraic variety. To study g-modules, one can instead study certain related D-modules on B. D-modules are sheaves of modules over the sheaves of differential operators. Goal To a complex semisimple Lie algebra g, one can attach its flag variety B. To study g-modules, one can instead study certain related D-modules on B. D-modules are sheaves of modules over the sheaves of differential operators. Goal To a complex semisimple Lie algebra g, one can attach its flag variety B. B is a smooth projective algebraic variety. D-modules are sheaves of modules over the sheaves of differential operators. Goal To a complex semisimple Lie algebra g, one can attach its flag variety B. B is a smooth projective algebraic variety. To study g-modules, one can instead study certain related D-modules on B. Goal To a complex semisimple Lie algebra g, one can attach its flag variety B. B is a smooth projective algebraic variety. To study g-modules, one can instead study certain related D-modules on B. D-modules are sheaves of modules over the sheaves of differential operators. I Can study them in local coordinates; I Can use geometric constructions like inverse and direct images; I Have geometric invariants like support or characteristic variety. Advantages of sheaves: I Can use geometric constructions like inverse and direct images; I Have geometric invariants like support or characteristic variety. Advantages of sheaves: I Can study them in local coordinates; I Have geometric invariants like support or characteristic variety. Advantages of sheaves: I Can study them in local coordinates; I Can use geometric constructions like inverse and direct images; Advantages of sheaves: I Can study them in local coordinates; I Can use geometric constructions like inverse and direct images; I Have geometric invariants like support or characteristic variety. I to introduce and study D-modules; I to show how D-modules are related to g-modules. References for most of what we will do can be found on Dragan Miliˇci´c'shomepage http://www.math.utah.edu/~milicic The aim of these lectures is: I to show how D-modules are related to g-modules. References for most of what we will do can be found on Dragan Miliˇci´c'shomepage http://www.math.utah.edu/~milicic The aim of these lectures is: I to introduce and study D-modules; References for most of what we will do can be found on Dragan Miliˇci´c'shomepage http://www.math.utah.edu/~milicic The aim of these lectures is: I to introduce and study D-modules; I to show how D-modules are related to g-modules. The aim of these lectures is: I to introduce and study D-modules; I to show how D-modules are related to g-modules. References for most of what we will do can be found on Dragan Miliˇci´c'shomepage http://www.math.utah.edu/~milicic n Let D(n) be the Weyl algebra of differential operators on C with polynomial coefficients. The algebra D(n) is generated by the partial derivatives @1;:::;@n and by the multiplication operators x1;:::; xn. These generators satisfy the commutation relations xi xj = xj xi ; @i @j = @j @i ; @i xj − xj @i = δij : The nontrivial relations come from the Leibniz rule: @i (xj P) = @i (xj )P + xj @i (P): The Weyl algebra The algebra D(n) is generated by the partial derivatives @1;:::;@n and by the multiplication operators x1;:::; xn. These generators satisfy the commutation relations xi xj = xj xi ; @i @j = @j @i ; @i xj − xj @i = δij : The nontrivial relations come from the Leibniz rule: @i (xj P) = @i (xj )P + xj @i (P): The Weyl algebra n Let D(n) be the Weyl algebra of differential operators on C with polynomial coefficients. These generators satisfy the commutation relations xi xj = xj xi ; @i @j = @j @i ; @i xj − xj @i = δij : The nontrivial relations come from the Leibniz rule: @i (xj P) = @i (xj )P + xj @i (P): The Weyl algebra n Let D(n) be the Weyl algebra of differential operators on C with polynomial coefficients. The algebra D(n) is generated by the partial derivatives @1;:::;@n and by the multiplication operators x1;:::; xn. The nontrivial relations come from the Leibniz rule: @i (xj P) = @i (xj )P + xj @i (P): The Weyl algebra n Let D(n) be the Weyl algebra of differential operators on C with polynomial coefficients. The algebra D(n) is generated by the partial derivatives @1;:::;@n and by the multiplication operators x1;:::; xn. These generators satisfy the commutation relations xi xj = xj xi ; @i @j = @j @i ; @i xj − xj @i = δij : The Weyl algebra n Let D(n) be the Weyl algebra of differential operators on C with polynomial coefficients. The algebra D(n) is generated by the partial derivatives @1;:::;@n and by the multiplication operators x1;:::; xn. These generators satisfy the commutation relations xi xj = xj xi ; @i @j = @j @i ; @i xj − xj @i = δij : The nontrivial relations come from the Leibniz rule: @i (xj P) = @i (xj )P + xj @i (P): The algebra D(1) is generated by x and @, with relation [@; x] = 1. A crucial remark is that D(1) cannot have any finite-dimensional modules. Namely, if M were a finite-dimensional D(1)-module, then the operator [@; x] on M would have trace 0, while the operator 1 would have trace dim M, a contradiction. Examples: D(1) A crucial remark is that D(1) cannot have any finite-dimensional modules. Namely, if M were a finite-dimensional D(1)-module, then the operator [@; x] on M would have trace 0, while the operator 1 would have trace dim M, a contradiction. Examples: D(1) The algebra D(1) is generated by x and @, with relation [@; x] = 1. Namely, if M were a finite-dimensional D(1)-module, then the operator [@; x] on M would have trace 0, while the operator 1 would have trace dim M, a contradiction. Examples: D(1) The algebra D(1) is generated by x and @, with relation [@; x] = 1. A crucial remark is that D(1) cannot have any finite-dimensional modules. Examples: D(1) The algebra D(1) is generated by x and @, with relation [@; x] = 1. A crucial remark is that D(1) cannot have any finite-dimensional modules. Namely, if M were a finite-dimensional D(1)-module, then the operator [@; x] on M would have trace 0, while the operator 1 would have trace dim M, a contradiction. An obvious example of a D(1)-module is the space of polynomials C[x], where elements of D(1) act by definition. This module is \smaller" and more interesting than D(1) with left multiplication. Another \small" example: truncated Laurent polynomials −1 C[x; x ]=C[x]. (These can be moved to any c 2 C.) Another way to describe an isomorphic module is as C[@], with @ · @i = @i+1; x · @i = −i@i−1: (\Fourier transform" of C[x].) Examples: D(1)-modules This module is \smaller" and more interesting than D(1) with left multiplication. Another \small" example: truncated Laurent polynomials −1 C[x; x ]=C[x]. (These can be moved to any c 2 C.) Another way to describe an isomorphic module is as C[@], with @ · @i = @i+1; x · @i = −i@i−1: (\Fourier transform" of C[x].) Examples: D(1)-modules An obvious example of a D(1)-module is the space of polynomials C[x], where elements of D(1) act by definition. Another \small" example: truncated Laurent polynomials −1 C[x; x ]=C[x]. (These can be moved to any c 2 C.) Another way to describe an isomorphic module is as C[@], with @ · @i = @i+1; x · @i = −i@i−1: (\Fourier transform" of C[x].) Examples: D(1)-modules An obvious example of a D(1)-module is the space of polynomials C[x], where elements of D(1) act by definition. This module is \smaller" and more interesting than D(1) with left multiplication. Another way to describe an isomorphic module is as C[@], with @ · @i = @i+1; x · @i = −i@i−1: (\Fourier transform" of C[x].) Examples: D(1)-modules An obvious example of a D(1)-module is the space of polynomials C[x], where elements of D(1) act by definition. This module is \smaller" and more interesting than D(1) with left multiplication. Another \small" example: truncated Laurent polynomials −1 C[x; x ]=C[x]. (These can be moved to any c 2 C.) Examples: D(1)-modules An obvious example of a D(1)-module is the space of polynomials C[x], where elements of D(1) act by definition. This module is \smaller" and more interesting than D(1) with left multiplication. Another \small" example: truncated Laurent polynomials −1 C[x; x ]=C[x]. (These can be moved to any c 2 C.) Another way to describe an isomorphic module is as C[@], with @ · @i = @i+1; x · @i = −i@i−1: (\Fourier transform" of C[x].) Since D(2) = D(1) ⊗ D(1), one can consider modules of the form M1 ⊗ M2, where Mi are D(1)-modules.
Details
-
File Typepdf
-
Upload Time-
-
Content LanguagesEnglish
-
Upload UserAnonymous/Not logged-in
-
File Pages408 Page
-
File Size-