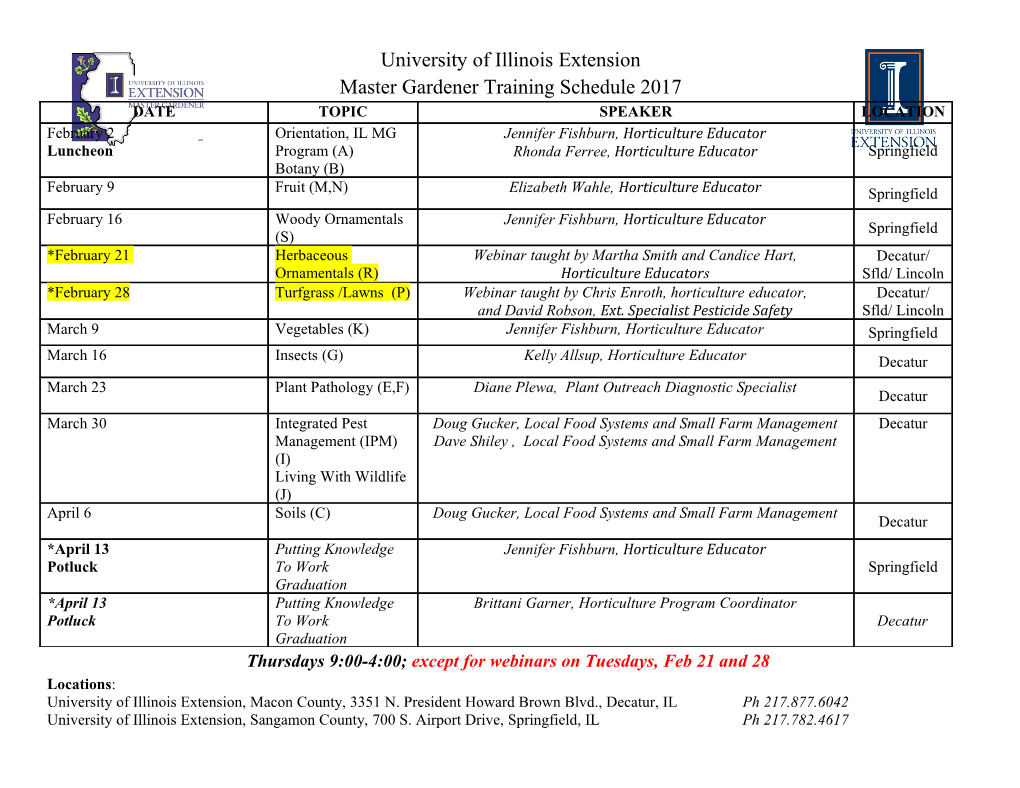
Colloids and Surfaces B: Biointerfaces 45 (2005) 154–161 Molecular mechanisms of phytochrome signal transduction in higher plants Li-Ye Chu a,b,1, Hong-Bo Shao a,b,c,∗,1, Mao-Yau Li a a Molecular Biology Laboratory, Bio-informatics College, Chongqing University of Posts & Telecommunications, Chongqing 400065, PR China b Biological Laboratory, Chemical Engineering College, Qingdao University of Science and Technology, Qingdao 266042, China c The State Key Laboratory of Soil Erosion and Dryland Farming on the Loess Plateau, The Centre of Soil and Water Conservation and Eco-environmental Research, The Chinese Academy of Sciences and Northwest Sci-tech University of Agriculture and Forestry, Yangling 712100, PR China Received 9 May 2005; accepted 28 May 2005 Abstract Phytochromes in higher plants play a great role in development, responses to environmental stresses and signal transduction, which are the fundamental principles for higher plants to be adapted to changing environment. Deep and systematic understanding of the phytochrome in higher plants is of crucial importance to molecular biology, purposeful improvement of environment in practice, especially molecular mechanism by which higher plants perceive UV-B stress. The last more than 10 years have seen rapid progress in this field with the aid of a combination of molecular, genetic and cell biological approaches. No doubt, what is the most important, is the application of Arabidopsis experimental system and the generation of various mutants regarding phytochromes (phy A–E). Increasing evidence demonstrates that phytochrome signaling transduction constitutes a highly ordered multidimensional network of events. Some phytochromes and signaling intermediates show light-dependent nuclear-cytoplasmic partitioning, which implies that early signaling events take place in the nucleus and that subcellular localization patterns most probably represent an important signaling control point. The main subcellular localization includes nucleus, cytosol and chloroplasts, respectively. Additionally, proteasome-mediated degradation of signaling intermediates most possibly function in concert with subcellular partitioning events as an integrated checkpoint. What higher plants do in this way is to execute accurate responses to the changes in the light environment on the basis of interconnected subcellular organelles. By integrating the available data, at the molecular level and from the angle of eco-environment, we should be able to construct a solid foundation for further dissection of phytochrome signaling transduction in higher plants. © 2005 Elsevier B.V. All rights reserved. Keywords: Phytochrome; Higher plants; Molecular biology; Signal transduction; An ordered multidimensional network 1. Introduction of events [1–4,30,37,42]. Light is one of the most impor- tant eco-environmental factors affecting higher plant growth, Phytochrome signal transduction in higher plants has often development and gene expression-control at the molecular been regarded as a non-spatially separated linear chain of level [16,37,41]. Higher plants respond to light in different events for the past 50 years. But a tight combination of ways ranging from simple signal perception and execution molecular, genetic and cell biological approaches have lead in single-cell prokaryotes to complicated signaling networks to current realization that phyrohrome signaling pathways in multi-cellular eukaryotes [1,29,40] (Bonioltti et al., 2002; are composed of a highly ordered multidimensional network Shao et al., 2004). Light not only functions as the most impor- tant energy source for photosynthesis, but higher plants have ∗ to monitor the light quality and quantity input for the sake of Corresponding author. Tel.: +86 29 87011190/23 62477654; executing the corresponding physiological and developmen- fax: +86 23 62460025/29 87012210. E-mail address: [email protected] (H.-B. Shao). tal responses. To reach this end, higher plants have evolved a 1 The authors contributed to this paper equally. complex set of photoreceptors, which include the blue/UV-A 0927-7765/$ – see front matter © 2005 Elsevier B.V. All rights reserved. doi:10.1016/j.colsurfb.2005.05.017 L.-Y. Chu et al. / Colloids and Surfaces B: Biointerfaces 45 (2005) 154–161 155 absorbing cryptochromes and the red/far-red light absorbing phytochromes [5]. The knowledge about the roles of phytochrome action is relatively complete, however, the insight into how the per- ceived light signals are transduced leading to morphological responses and altered gene expression patterns has remained somewhat sparse [1]. More recently, the isolation of mutants Fig. 1. Structural features of phytochrome B protein, indicating the positions of the chromophore attachment site, serine phosphorylation sites, histidine disrupted in genes encoding phytochrome signaling interme- kinase-related domains (HKRD) and the two PAS domains [1,22,36,37]. diates have identified both positive and negative components of these phytochrome signaling pathways. In addition, using protein–protein interaction technology, such as yeast two-hybrid and in vitro pull-down assays, there have been red reversible plant responses. It has since been clear that exciting advances in understanding the plethora of physical phytochromes belong to closely related family of photore- interactions that take place not only between phytochrome ceptors, the apoproteins of which are encoded by a small and early signaling intermediates but also between bona fide family of divergent genes. Arabidopsis thaliana has five dis- signaling components [5–11]. An emerging viewpoint from crete apophytochrome-encoding genes, PHYA-PHYE, iso- the available data is that phytachrome signal transduction in lated and sequenced [23–25]. Arabidopsis PHYB and PHYD higher plants is a highly ordered but yet complex network polypeptides are approximately 80% identical and are more of events with different branches of the signaling network closely related to PHYE than they are to either PHYA or spatially separated into different subcellular compartments PHYC (approximately 80% identity) [27,28,37]. Counter- (Xiong et al., 2001) [22]. Moreover, several other nuclear parts of PHTA, PHYB and other PHY genes are present in phytochrome signaling components have been identified most, it not all, higher plants. clearly implying that the nucleus is a hot-spot for early All of the higher plant phytochromes appear to share the signaling events [1,5]. Nowadays, there have been a larger same basic structure, consisting of a dimmer of identical number of components that we need to integrate at the whole 124 kDa polypeptides. Each monomer carries a single cova- cell level to further understand phytochrome signal transduc- lently linked linear tetrapyrrole chromophore (phytochromo- tion. It is evident that many organelles are actively involved bilin), attached via a thieother bond to a conserved cysteine in phytochrome signaling and that these organelles are residue in the N-terminal globular domain of the protein. interconnected [12,13,26]. It is also clear that the subcellular The C-terminal domain encompasses two histidine kinase- partitioning of both phytochrome and signaling intermediates related domains (HRKD) and two motifs with homology to provides an elegant controling mechanism. Likewise, the PAS (PER-ARNT-SIM) domains [9,10,14,37]. PAS domains finely tuned degradation of signaling pathway components are present in various signal transduction molecules which by the 26s proteasome is clearly an important checkpoint sense environmental signals, such as light conditions, oxy- [14,15,30–32]. Additionally, the multitude of interactions gen levels and redox potential [1]. They may also mediate that occur among phytochrome signaling pathways is very protein–protein interaction (Fig. 1). The amino terminal half attractive and these interactions depend on controlled subcel- of phytochromes can be considered as a light sensing dor- lular partitioning events [1,17,18]. This paper focuses on the main whist the carboxyl-terminal half can be regarded as the molecular biology of phytochrome signaling transduction regulatory domain (Fig. 1). in higher plants in terms of components of its different Higher plant phyrochrome can be classified into two pathways, the overall phytochrome signaling network and groups based on the stability in light: type I (phy A) occurs main existing problems in this frontier area. It is an accepted in etiolated tissues in large quantities and is subject to a fact that one of the keypoints to further dissect phytochrome high turnover, i.e., is light labile and type II (phy B–phy signal transduction is to use the spectrum of molecular E), are light stable. Higher plant phytochromes undergo and biochemical tools available, not only to isolate new photoconversion between two stable states: the red light signaling components, but also to integrate the pool of phy- absorbing form (Pr, synthesized in the dark) and the farred tochrome knowledge in a cellular and molecular biological light absorbing form (Pfr). This Pr-to-Pfr transition is due context. to a light induced double bond rotation in the chromophore and rearrangements of the protein backbone of the apopro- tein. For most responses Pfr is considered to be the bio- 2. Basic structure and functions of phytochromes in logically active form. The Pr forms of (at least some) higher plants phytochromes of higher plants localize to the cytoplasm, whereas a proportion of the Pfr (active) isofoms localize 2.1.
Details
-
File Typepdf
-
Upload Time-
-
Content LanguagesEnglish
-
Upload UserAnonymous/Not logged-in
-
File Pages8 Page
-
File Size-