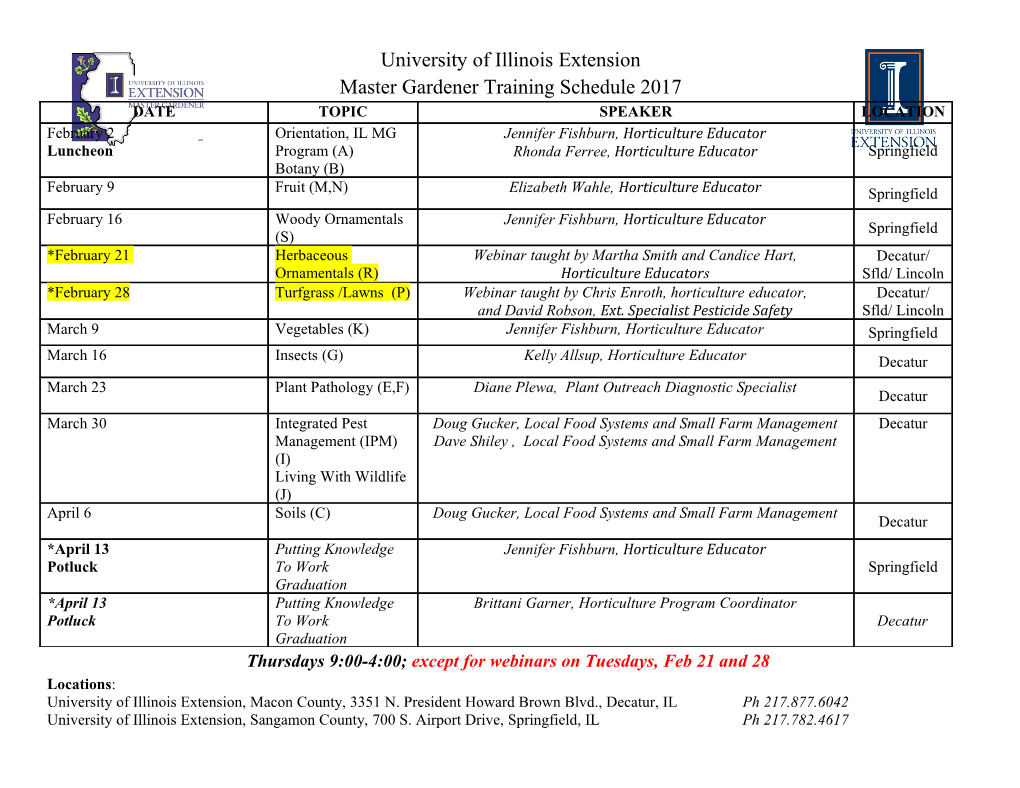
J Mol Evol (2004) 59:250–257 DOI: 10.1007/s00239-004-2619-6 Origin and Evolution of Tandem Repeats in the Mitochondrial DNA Control Region of Shrikes (Lanius spp.) Nicholas I. Mundy,1 Andreas J. Helbig2 1 Department of Zoology, University of Cambridge, Downing Street, Cambridge, CB2 3EJ, UK 2 Vogelwarte Hiddensee, Universitat Greifswald, D-18565 Kloster/Hiddensee, Germany Received: 25 September 2003 / Accepted: 12 February 2004 [Reviewing Editor: Martin Kreitman] Abstract. The origin and evolution of a 128-bp number of taxa (reviewed in Hoelzel 1993; Rand 1993; tandem repeat in the mtDNA control region of Lunt et al. 1998). In birds, control region tandem re- shrikes (Lanius: Aves) were investigated. The tandem peats have been documented at the sequence level in repeat is present in only two species, L. excubitor and waders, gulls, auks, shrikes, penguins, and eagles L. ludovicianus. In contrast to the variation in repeat (Wenink et al. 1994; Berg et al. 1995; Mundy et al. number in L. ludovicianus, all individuals of three 1996; Ritchie and Lambert 2000; Va¨ li 2002). These subspecies of L. excubitor had three repeats. Com- repeats can be categorized according to size of the parative analysis suggests that a short direct repeat, basic repeat unit and the position of the array within and a secondary structure including the tandem repeat the control region (Hoelzel 1993). Interest in repeats and a downstream inverted repeat, may be important has focused on the light they shed on mechanisms of in the origin of the tandem repeat by slipped-strand mtDNA mutation and turnover and on the practical mispairing and its subsequent turnover. Homogeni- use of repeats as markers in population genetics. zation of repeat sequences is most simply explained by The origin of mtDNA tandem repeats is generally expansion and contraction of the repeat array. Sur- thought to involve slipped-strand mispairing during prisingly, mtDNA sequences from L. excubitor were mtDNA replication (Levinson and Gutman 1987; found to be paraphyletic with respect to L. ludovici- Buroker et al. 1990; Hayasaka et al. 1991; Madsen anus. These results show the utility of a comparative et al. 1993; Fumagalli et al. 1996; Broughton and analysis for insights into the evolutionary dynamics of Dowling 1994; Wilkinson et al. 1997). Other mecha- mtDNA tandem repeats. nisms for repeat generation, such as intermolecular recombination, are considered rare (reviewed in Ro- Key words: Mitochondrial DNA — Control region kas et al. 2003). However, the details of how slipped- — Tandem repeats strand mispairing works are poorly understood and there has been little work on why repeats form in some lineages and not others. As well as being of interest to control region repeats themselves, such mechanisms are also relevant to other examples of Introduction mtDNA turnover. For example, one favored hy- pothesis for the generation of mitochondrial gene Tandemly repeated DNA in the control region of the order rearrangements involves a large duplication mitochondrial DNA is reported from an ever-growing event followed by gene loss (Macey et al. 1997). In previous work we demonstrated that there is a 128-bp tandem repeat in the control region of the Correspondence to: Nicholas I. Mundy; email: [email protected] loggerhead shrike (Lanius ludovicianus) (Mundy et al. 251 1996) (Fig. 1A). All individuals of this species either have two repeats, have three repeats, or are hetero- plasmic for two and three repeats. In contrast, the one other species of shrike examined (L. collaris) did not have the repeat. The 128-bp tandem repeat in the loggerhead shrike falls into the relatively small cate- gory of large repeats that are present in a 3¢ position in the light strand of the control region. Other ex- amples in this category include repeat arrays in rab- bits (Mignotte et al. 1990), macaques (Hayasaka et al. 1991), minnows (Broughton and Dowling 1994), redfish (Bentzen et al. 1998), and crocodiles (Ray and Densmore 2003). An important feature of these tan- dem repeat arrays is that since they presumably occur Fig. 1. A Structure of mtDNA in species with (L. ludovicianus; top) and without (L. collaris; bottom) the 128-bp tandem repeat. 3¢ to the origin of heavy strand replication (OH) they CSB-1 is conserved sequence block 1. DLL2 and FTPH2 are the do not form part of the D-loop, and thus mechanisms primers used in this study. B Model of tandem repeat formation of slipped-strand mispairing that involve the D-loop (Mundy et al. 1996). The light strand is the lower strand. Toward the end of heavy-strand replication, the leading strand spontane- (e.g., Buroker et al. 1990) are not relevant to their ously denatures (top). Stem-loop formation by the inverted repeat origin and expansion/contraction. occurs and the strand reanneals onto the direct repeat sequence Other sequence motifs in and around the tandem (middle). Continued replication generates the tandem repeat (bot- repeat led to the proposal of a model of how the tom). tandem repeat arose by slipped-strand mispairing in shrikes, toward the end of heavy strand replication collected during banding procedures. Abbreviations follow genus, (Mundy et al. 1996; Fig. 1B), which was a variation species, and subspecies for species with more than one subspecies (e.g., Lei for Lanius excubitor invictus) and genus, species for spe- of a model originally proposed by Broughton and cies with no subspecies (e.g., Lco for Lanius collaris). The single Dowling (1994). In the model, short direct repeats exception involves L. ludovicianus mearnsi and L. l. gambeli, whose were proposed to be important for reannealing fol- haplotypes are labeled Llu1, Llu2, and Llu3, to be consistent with lowing strand slippage, and an inverted repeat 3¢ to nomenclature used in the previous study (Mundy et al. 1996). the tandemly repeated region was proposed to form a stable stem loop structure following slippage that Laboratory Methods stabilized the slipped strand to promote reannealing to a different position. However, data from two All laboratory procedures closely followed those used previously species were insufficient to critically appraise this (Mundy et al. 1996). Total genomic DNA was extracted from single model. feather shafts using Chelex and from blood using a salting-out procedure. Primers used to amplify the region containing tandem Key questions to test the model are: In the line- repeats were DLL2 (5¢ ATGCACTTTTACCCCATTCATGGTGG age(s) in which the tandem repeat originated, were 3¢) and FTPH2 (5¢ CCATCTTGACATCTTCAGTGCCATGC 3¢) the direct repeats present? Were the inverted repeats (see Fig. 1A for positions). Polymerase chain reaction (PCR) am- present? and Were predicted free energies of second- plifications were performed in a Hybaid thermal cycler in a 25-ll ary structures comparatively high? Absence of any of total volume containing 0.5–1.0 units Taq polymerase (Perkin–El- mer), 1· PCR buffer, a 50 lM concentration each dNTP, 1.5 mM these would lead to rejection of the model. In the MgCl2, and 25 lg bovine serum albumin (Fraction V, Sigma), and present study we have obtained an expanded dataset 10–100 ng template DNA. Cycling parameters were as follows: 1 · of control region sequences from 8 species and 14 94°C, 3 min; 30–40 · 94°C, 30 s; 50–60°C, 60 s, 72°C, 90 s; and 1 · subspecies of shrikes, including the great grey shrike 72°C, 10 min. Different samples required different annealing tem- (L. excubitor), which is generally considered to be the peratures for optimization. Direct double-stranded sequencing of PCR products was performed with Sequenase (USB) and 35S- sister species of L. ludovicianus. The results show that dATP. Products of sequencing reactions were separated on 8% the tandem repeat is only present in L. excubitor and polyacrylamide gels (Long Ranger; J. T. Baker). Dried gels were L. ludovicianus, support the model of repeat origi- exposed to X-ray film (X-Omat; Kodak Eastman) for 1–7 days. nation, and shed light on mechanisms of repeat Sequences were read by eye. turnover. DNA Sequence Analysis Materials and Methods Sequences were aligned using Clustal X (Higgins and Sharp 1989). A control region sequence from a rook (Corvus frugilegus) was used Samples as the closest available outgroup (GenBank Accession No. Y18522 [Ha¨ rlid and Arnason 1999]). Phylogenetic analyses were performed Species and subspecies sampled in the study are shown in Table 1. in PAUP* (Swofford 1999). Neighbor-joining trees were con- Samples from L. ludovicianus and L. excubitor invictus were structed using HKY distances. Branch support in neighbor-joining plucked feathers; those from other species were blood samples and parsimony was assessed using 1000 bootstrap replicates. 252 Table 1. Shrike taxa sampled in this study Taxon Origin Lab ID Loggerhead shrike Lanius ludovicianus mearnsi San Clemente I., USA Haplotypes Llu1, Llu2 (Mundy et al. 1996)a L. l. gambeli California, USA Haplotypes Llu2, Llu3 (Mundy et al. 1996)a L. l. excubitorides Alberta, Canada Lle6 Great grey shrike L. e. excubitor Germany Lee1, Lee2, Lee3 Northern shrike L. e. invictus Oregon, USA Lei1, Lei2, Southern grey shrike L. e. meridionalis Spain Lem1, Lem2, Lem3 Fiscal shrike L. collaris Guinea Lco1 (Mundy et al. 1996) Woodchat shrike L. s. senator Italy Lss1, Lss3 L. s. badius Italy Lsb4 L. s. niloticus Israel Lsn5 Red-backed shrike L. c. collurio Italy Lcc3, Lcc6 Isabelline shrike L. isabellinus Mongolia Lis1, Lis2 Masked shrike L. n. nubicus Israel Lnn1 Long-tailed shrike L. s. schach Hong Kong Lsc1, Lsc2 a Haplotypes Llu1, Llu2, and Llu3 were obtained in the previous study (Mundy et al. 1996), from numerous individuals of L. l. mearnsi and L. l. gambeli, and these designations are retained here for ease of comparison.
Details
-
File Typepdf
-
Upload Time-
-
Content LanguagesEnglish
-
Upload UserAnonymous/Not logged-in
-
File Pages8 Page
-
File Size-