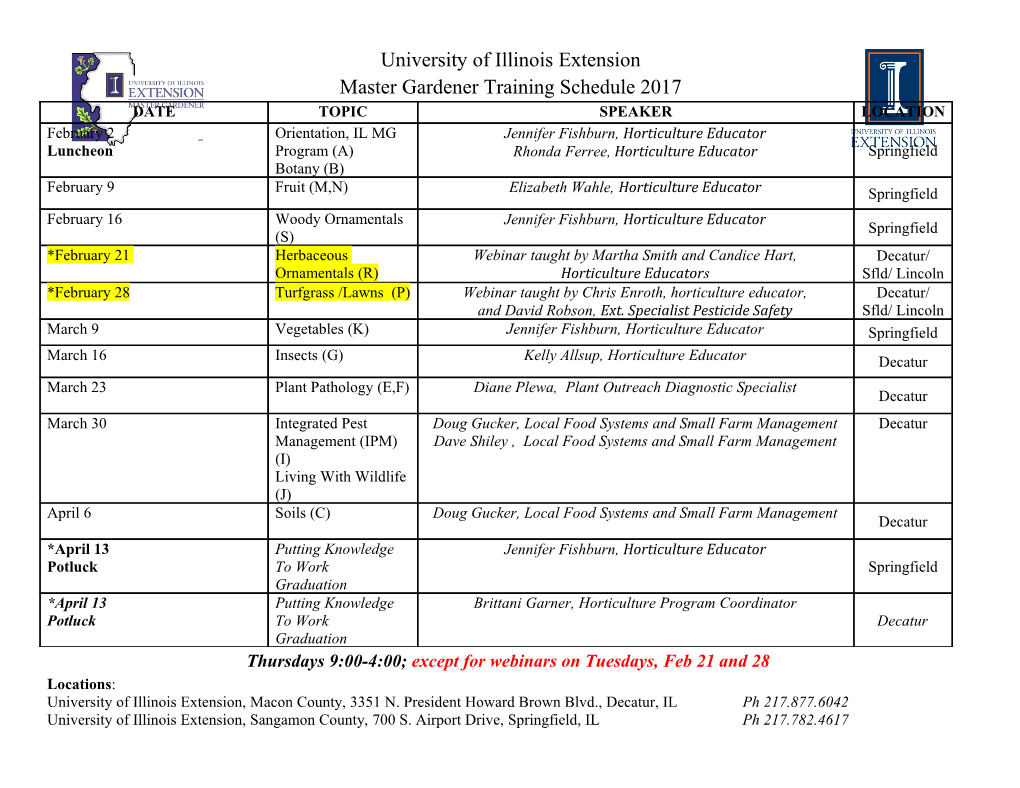
Lecture 22 Relativistic Quantum Mechanics Background Why study relativistic quantum mechanics? 1 Many experimental phenomena cannot be understood within purely non-relativistic domain. e.g. quantum mechanical spin, emergence of new sub-atomic particles, etc. 2 New phenomena appear at relativistic velocities. e.g. particle production, antiparticles, etc. 3 Aesthetically and intellectually it would be profoundly unsatisfactory if relativity and quantum mechanics could not be united. Background When is a particle relativistic? 1 When velocity approaches speed of light c or, more intrinsically, when energy is large compared to rest mass energy, mc2. e.g. protons at CERN are accelerated to energies of ca. 300GeV (1GeV= 109eV) much larger than rest mass energy, 0.94 GeV. 2 Photons have zero rest mass and always travel at the speed of light – they are never non-relativistic! Background What new phenomena occur? 1 Particle production e.g. electron-positron pairs by energetic γ-rays in matter. 2 Vacuum instability: If binding energy of electron 2 4 Z e m 2 Ebind = > 2mc 2!2 a nucleus with initially no electrons is instantly screened by creation of electron/positron pairs from vacuum 3 Spin: emerges naturally from relativistic formulation Background When does relativity intrude on QM? 1 When E mc2, i.e. p mc kin ∼ ∼ 2 From uncertainty relation, ∆x∆p > h, this translates to a length h ∆x > = λ mc c the Compton wavelength. 3 for massless particles, λc = , i.e. relativity always important for, e.g., photons. ∞ Relativistic quantum mechanics: outline 1 Special relativity (revision and notation) 2 Klein-Gordon equation 3 Dirac equation 4 Quantum mechanical spin 5 Solutions of the Dirac equation 6 Relativistic quantum field theories 7 Recovery of non-relativistic limit Special relativity (revision and notation) Space-time is specified by a 4-vector A contravariant 4-vector x =(x µ) (x 0, x 1, x 2, x 3) (ct, x) ≡ ≡ ν transformed into covariant 4-vector xµ = gµν x by Minkowskii metric 1 µν 1 µν µ µ (gµν ) = (g )= − , g gνλ = g δ , 1 λ ≡ λ − 1 − Scalar product: x y = x y µ = x µy ν g = x µy · µ µν µ Special relativity (revision and notation) Lorentz group: consists of linear transformations, Λ, preserving µ µ µ ν x y, i.e. for x x ! =Λ x = x y · %→ ν · µ ν µ ν α β α β x ! y ! = g x ! y ! = g Λ Λ x y = g x y · µν µν α β αβ = gαβ ' () * e.g. Lorentz transformation along x1 γ γv/c γv/c − γ 1 Λµ = − ,γ = ν 10 (1 v 2/c2)1/2 01 − Special relativity (revision and notation) 4-vectors classified as time-like or space-like x 2 =(ct)2 x2 − 1 forward time-like: x 2 > 0, x 0 > 0 2 backward time-like: x 2 > 0, x 0 < 0 3 space-like: x 2 < 0 Special relativity (revision and notation) Lorentz group splits up into four components: 1 Every LT maps time-like vectors (x 2 > 0) into time-like vectors 2 0 Orthochronous transformations Λ 0 > 0, preserve forward/backward sign 3 Proper: det Λ= 1 (as opposed to 1) − 4 Group of proper orthochronous transformation: +↑ – subgroup of Lorentz group – excludes time-reversalL and parity 1 1 − 1 1 T = , P = 1 − 1 − 1 1 − 5 Remaining components of group generated by ↓ = T +↑ , ↑ = P +↑ , +↓ = TP +↑ . L− L L− L L L Special relativity (revision and notation) 1 Special relativity requires theories to be invariant under LT or, more generally, Poincar´etransformations: x µ Λµ x ν + aµ → ν 2 Generators of proper orthochronous transformations, Λ +↑ , can be reached by infinitesimal transformations ∈L Λµ = δµ + ωµ ,ωµ 1 ν ν ν ν ( µ ν 2 ! gµν Λ αΛ β = gαβ + ωαβ + ωβα + O(ω ) = gαβ i.e. ω = ω , ω has six independent components αβ − βα αβ ↑ has six independent generators: three rotations and three boosts L+ µ 3 covariant and contravariant derivative, chosen s.t. ∂µx =1 ∂ 1 ∂ ∂ 1 ∂ ∂ = = , ,∂µ = = , . µ ∂x µ c ∂t ∇ ∂x c ∂t −∇ + , µ + , 1 ∂2 4 d’Alembertian operator: ∂2 = ∂ ∂µ = 2 µ c2 ∂t2 −∇ Relativistic quantum mechanics: outline 1 Special relativity (revision and notation) 2 Klein-Gordon equation 3 Dirac equation 4 Quantum mechanical spin 5 Solutions of the Dirac equation 6 Relativistic quantum field theories 7 Recovery of non-relativistic limit Klein-Gordon equation Klein-Gordon equation How to make wave equation relativistic? According to canonical quantization procedure in NRQM: ˆ µ µ pˆ = i! , E = i!∂t , i.e. p (E/c, p) pˆ − ∇ ≡ %→ transforms as a 4-vector under LT What if we apply quantization procedure to energy? pµp =(E/c)2 p2 = m2c2, m rest mass µ − − 2 4 2 2 1/2 2 4 2 2 2 1/2 E(p) = + m c + p c i!∂t ψ = m c ! c ψ %−→ − ∇ Meaning of- square root?. Taylor expansion: / 0 2 2 4 2 2 2 ! ! ( ) i!∂t ψ = mc ψ ∇ ψ ∇ ψ + − 2m − 8m3c2 ··· i.e. time-evolution of ψ specified by infinite number of boundary conditions non-locality, and space/time asymmetry – suggests that this equation%→ is a poor starting point... Klein-Gordon equation Alternatively, apply quantization to energy-momentum invariant: 2 2 2 2 4 2 2 2 2 2 2 4 E = p c + m c , ! ∂ ψ = ! c + m c ψ − t − ∇ - . 2π mc Setting kc = = , leads to Klein-Gordon equation, λc ! 2 2 ∂ + kc ψ =0 - . Klein-Gordon equation is local and manifestly Lorentz covariant. Invariance of ψ under rotations means that, if valid at all, Klein-Gordon equation limited to spinless particles But can ψ 2 be interpreted as probability density? | | Klein-Gordon equation: Probabilities Probabilities? Take lesson from non-relativistic quantum mechanics: Schrodinger eqn. c.c. !2 2 !2 2 ψ∗ i!∂t + ∇ ψ =0, ψ i!∂t + ∇ ψ∗ =0 2m − 2m +) *' , ( ) + *' , ( 2 ! i.e.∂ ψ i (ψ∗ ψ ψ ψ∗)=0 t | | − 2m ∇· ∇ − ∇ cf. continuity relation – conservation of probability: ∂ ρ + j =0 t ∇· 2 ! ρ = ψ , j = i (ψ∗ ψ ψ ψ∗) | | − 2m ∇ − ∇ Klein-Gordon equation: Probabilities 1 2 2 2 Applied to KG equation: ψ∗ ∂ + k ψ =0 c2 t −∇ c + , 2 2 2 ! ∂t (ψ∗∂t ψ ψ∂t ψ∗) ! c (ψ∗ ψ ψ ψ∗)=0 − − ∇· ∇ − ∇ cf. continuity relation – conservation of probability: ∂ ρ + j = 0. t ∇· ! ! ρ = i (ψ∗∂ ψ ψ∂ ψ∗) , j = i (ψ∗ ψ ψ ψ∗) 2mc2 t − t − 2m ∇ − ∇ µ µ With 4-current j =(ρc, j), continuity relation ∂µj = 0. i.e. Klein-Gordon density is the time-like component of a 4-vector. Klein-Gordon equation: viability? But is Klein-Gordon equation acceptable? ! Density ρ = i (ψ∗∂ ψ ψ∂ ψ∗) is not positive definite. 2mc2 t − t Klein-Gordon equation is not first order in time derivative therefore we must specify ψ and ∂t ψ everywhere at t = 0. Klein-Gordon equation has both positive and negative energy solutions. Could we just reject negative energy solutions? Inconsistent – local interactions can scatter between positive and negative energy states ∂2 + k2 ψ = F (ψ) self interaction c − 2 2 (∂-+ iqA/!.c) + kc ψ =0 interaction with EM field 1 2 Relativistic quantum mechanics: summary When the kinetic energy of particles become comparable to rest mass energy, p mc particles enter regime where relativity intrudes on quantum mechanics.∼ At these energy scales qualitatively new phenomena emerge: e.g. particle production, existence of antiparticles, etc. By applying canonical quantization procedure to energy-momentum invariant, we are led to the Klein-Gordon equation, 2 2 (∂ + kc )ψ =0 λc ! where λ = = denotes the Compton wavelength. 2π mc However, the Klein-Gordon equation does not lead to a positive definite probability density and admits positive and negative energy solutions – these features led to it being abandoned as a viable candidate for a relativistic quantum mechanical theory. Lecture 23 Relativistic Quantum Mechanics: Dirac equation Relativistic quantum mechanics: outline 1 Special relativity (revision and notation) 2 Klein-Gordon equation 3 Dirac equation 4 Quantum mechanical spin 5 Solutions of the Dirac equation 6 Relativistic quantum field theories 7 Recovery of non-relativistic limit Dirac Equation Dirac placed emphasis on two constraints: 1 Relativistic equation must be first order in time derivative (and therefore proportional to ∂ =(∂ /c, )). µ t ∇ 2 Elements of wavefunction must obey Klein-Gordon equation. Dirac’s approach was to try to factorize Klein-Gordon equation: (∂2 + m2)ψ = 0 (where henceforth we set ! = c = 1) ( iγν ∂ m)(iγµ∂ m)ψ =0 − ν − µ − i.e. withp ˆµ = i∂µ (γµpˆ m) ψ =0 µ − Dirac Equation (γµpˆ m) ψ =0 µ − Equation is acceptable if: 1 ψ satisfies Klein-Gordon equation, (∂2 + m2)ψ = 0; 2 there must exist 4-vector current density which is conserved and whose time-like component is a positive density; 3 ψ does not have to satisfy any auxiliary boundary conditions. µ From condition (1) we require (assuming [γ , pˆν ] = 0) 0=(γν pˆ + m)(γµpˆ m) ψ =(γν γµ pˆ pˆ m2)ψ ν µ − ν µ − (γν γµ+γµγν )/2 1 = γν ,γµ pˆ pˆ m2 ψ =(g'νµ()pˆ*pˆ m2)ψ =(p2 m2)ψ 2{ } ν µ − ν µ − − + , i.e. obeys Klein-Gordon if γµ,γν γµγν + γν γµ =2g νµ { γµ, and}≡ therefore ψ, can not be scalar. ⇒ Dirac Equation: Hamiltonian formulation (γµpˆ m) ψ =0, γµ,γν =2g νµ µ − { } To bring Dirac equation to the form i∂t ψ = Hˆ ψ, consider γ0(γµpˆ m)ψ = γ0(γ0pˆ γ pˆ m)ψ =0 µ − 0 − · − Since (γ0)2 1 γ0,γ0 = g 00 = I, ≡ 2 { } γ0(γµpˆ m)ψ = i∂ ψ γ0γ pˆψ mγ0ψ =0 µ − t − · − i.e.
Details
-
File Typepdf
-
Upload Time-
-
Content LanguagesEnglish
-
Upload UserAnonymous/Not logged-in
-
File Pages65 Page
-
File Size-